Exploring Chemistry Experiments: Foundations and Applications
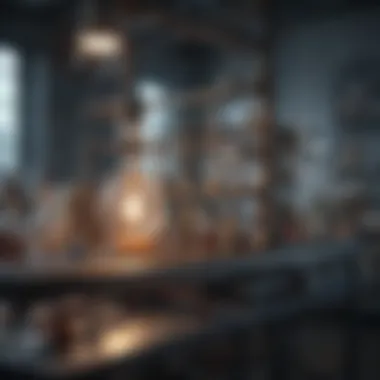
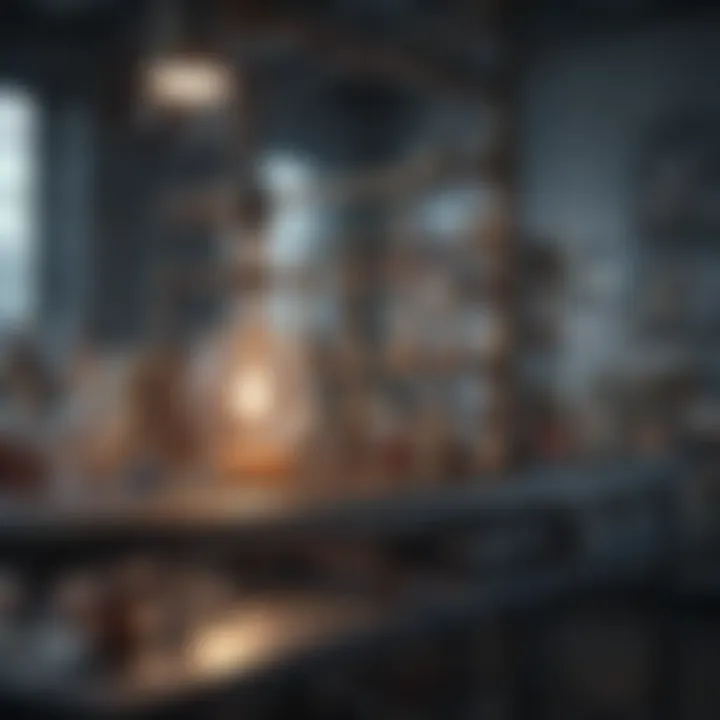
Intro
Chemistry experiments serve as the backbone of scientific inquiry, allowing us to peel back the layers of the natural world. These experiments range from simple reactions in a high school lab to complex studies that require cutting-edge technology. Understanding the foundations of these experiments helps to bridge the gap between theoretical principles and practical applications.
From the early days, when alchemists dabbled in transformations of materials, to today's sophisticated methodologies, chemistry has evolved tremendously. This article aims to take a closer look at the fundamental principles that underpin chemistry experiments and their significance in various scientific fields. By contextualizing these methodologies within historical developments, we can appreciate the transition into modern experimental practices and their relevance.
As we go deeper, we’ll uncover the innovative techniques that propel the future of chemistry. With a focus on not only what's been achieved but also the avenues left unexplored, this exploration promises to enrich your understanding of chemistry experimentation in profound ways.
Foreword to Chemistry Experiments
Chemistry experiments serve as the bedrock of scientific inquiry in the field. They enable us to translate abstract concepts into observable reality. Experiments are not just procedures carried out in labs; they embody the essence of scientific exploration, where hypotheses are rigorously tested and new knowledge is forged. This article aims to shine a spotlight on the pivotal role of chemistry experiments, a crucial element that guides us through the interconnected web of theoretical and practical chemistry.
The Importance of Experiments in Chemistry
Experiments are the lifeblood of chemistry. They provide a structured way for scientists to validate theories, offering tangible evidence to support or refute scientific ideas. Imagine a chemist hypothesizing about a compound's behavior. Without experimentation, that hypothesis remains mere speculation.
Through controlled conditions and variable manipulation, chemists can observe chemical reactions and their outcomes, allowing for precise conclusions. This practice not only fosters deeper understanding but also drives innovation in countless applications.
The adaptability and versatility of experiments open up pathways to numerous benefits:
- Validation of Theories: Experiments serve as the tool to confirm or challenge established scientific views, essential for scientific progress.
- Innovation and Discovery: Many groundbreaking inventions stem from experimentation, leading to advancements in various fields, such as pharmaceuticals and sustainable materials.
- Education and Training: Hands-on experiments in educational settings encourage critical thinking and problem-solving skills, necessary for future scientists.
In this way, the significance of experiments in chemistry transcends mere knowledge acquisition, helping professionals and students alike to cultivate a robust scientific mindset.
Historical Overview of Chemistry Experiments
To truly appreciate the foundations of modern chemistry experiments, it is crucial to take a step back in time. The history of chemistry is dotted with remarkable milestones, each representing a significant shift in our understanding of matter and reactions.
From the alchemical practices of ancient civilizations—where the quest to transform base metals into gold symbolized the potential for change, albeit often through a spiritual lens—to the scientific rigor championed by figures like Antoine Lavoisier, who is widely regarded as the father of modern chemistry, the evolution of experimentation is profound.
In the 18th century, Lavoisier laid the groundwork for chemical experimentation by introducing systematic methodologies, such as careful measurement and controlled conditions. His work illustrated that conservation of mass in chemical reactions is vital; this paved the way for more precise scientific inquiry.
Throughout the 19th and 20th centuries, new theories and technologies emerged, each revolutionizing how experiments were conducted. With the advent of spectroscopy and chromatography, chemists gained new insights into the structures and behaviors of molecules, leading to transformative discoveries. The progression of chemistry experiments reflects broader societal changes and technological advancements, forming a rich tapestry of knowledge that continues to grow.
"The process of learning through experimentation is what keeps the flame of pursuit burning in the scientific community."
As we further delve into the boundaries of chemistry experiments, it is clear that this historical perspective not only enriches our understanding of the discipline but also informs the ethical and methodological considerations that guide today's research.
Key Concepts in Chemical Experimentation
In the landscape of scientific inquiry, key concepts in chemical experimentation serve as the bedrock upon which sound research is built. They formulate the backbone of any successful chemical study, guiding researchers through a structured process that begins with insightful questions and culminates in accurate conclusions. It's vital for students, educators, and professionals to grasp these concepts, not just for academic purposes, but also to enable them to tackle real-world problems effectively.
Research efforts that lack a solid understanding of these concepts often drift aimlessly, leading to conclusions that may be misleading or entirely wrong. Proper grasp of experimentation fundamentals—the hypothesis, testing variables, and data interpretation—holds significant implications for both the validity of results and their applicability in broader contexts. Additionally, these elements strike a balance between creativity and rigor, ensuring results are both imaginative and reproducible.
Hypothesis Formation and Testing
Hypothesis formation and testing is where the journey of experimentation begins. A well-crafted hypothesis is more than just a best guess; it serves as a specific and testable prediction about what will happen under certain conditions. For instance, if a researcher hypothesizes that increasing temperature will accelerate the rate of a chemical reaction, this sets the stage for definitive observation and assessment.
To ensure a hypothesis remains relevant, it often undergoes revision—a process akin to sculpting a block of marble until the masterpiece within emerges. Here are some common steps in hypothesis testing:
- Identifying a Problem: Observations spark questions worthy of investigation.
- Formulating a Hypothesis: Establishing a clear, concise, and testable statement.
- Experimentation: Conducting experiments to collect data that affirms or refutes the hypothesis.
- Analysis: Evaluating the data objectively, ensuring biases do not warp the interpretation.
- Conclusions: Drawing conclusions that accurately reflect the experimental outcomes.
Testing a hypothesis isn't just about finding what you expect; it's about being open to discovering the unexpected. As the renowned physicist Richard Feynman once said,
"The first principle is that you must not fool yourself—and you are the easiest person to fool."
Thus, embracing both outcomes allows researchers to contribute to the collective body of scientific knowledge.
Variables in Chemical Experiments
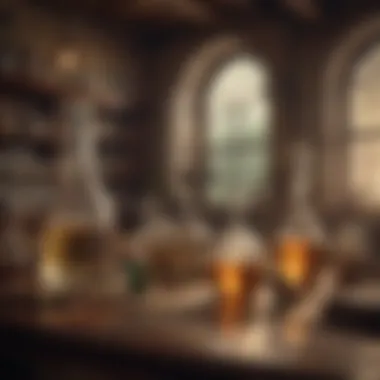
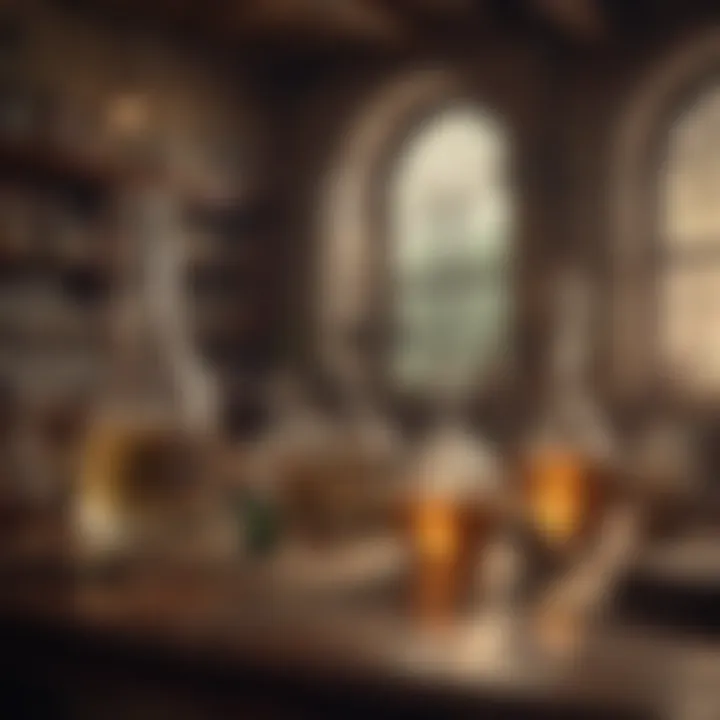
Variables are crucial to understanding the nuances of chemical experiments. Each experiment is a dance of many elements, especially the variables that can influence the outcome. Variables generally fall into three broad categories:
- Independent Variables: The ones that researchers manipulate or change. For example, if you're studying the effect of pH on enzyme activity, the pH level would be the independent variable.
- Dependent Variables: These are the outcomes that researchers measure. Continuing with the enzyme example, the rate of reaction would serve as the dependent variable, dependent on the pH you set.
- Controlled Variables: Factors kept constant to ensure the experiment is fair. For instance, temperature or concentration should remain consistent to avoid skewed results.
It’s key to maintaining clarity of thought about these factors when designing an experiment. A failure to control certain variables can lead to spurious correlations and complicate the interpretation of results. In the wild world of chemistry, a small oversight can lead to a domino effect of inaccurate conclusions.
By closely monitoring variables, researchers can paint a clearer picture of causal relationships, ultimately allowing for more robust interpretations of the data gathered. Overall, understanding these fundamental concepts creates a framework for reliable and reproducible results, a cornerstone of scientific integrity.
Methodologies in Chemistry Experiments
When delving into the field of chemistry, one soon learns that methodologies form the backbone of scientific inquiry. Understanding these methodologies allows researchers not only to conduct experiments more effectively but also to appreciate the broader implications of their findings. In this section, we’ll explore the distinctive methodologies, detailing how they shape experimental outcomes and contribute to reliable scientific advancement. The intricate dance between diverse methodologies is what turns theoretical notions into practical applications. Without a solid grasp of these methods, any experimentation might as well be akin to shooting in the dark.
Qualitative vs. Quantitative Analysis
In any chemical experiment, distinguishing between qualitative and quantitative analysis is essential. Qualitative analysis seeks to identify the presence of particular substances. A simple example would be testing soil for the presence of certain metals. Here, we aren't concerned with how much of each metal is present; rather, the focus is purely on determining whether they exist at all.
Conversely, quantitative analysis delves into the measurement and quantification of these substances. This might involve calculating the exact concentration of lead in a sample. Quantitative analysis is vital for regulatory compliance and to mitigate potential risks in various industries. Combining both forms of analysis often yields a fuller picture of chemical phenomena, allowing for enhanced strategic decisions in research and applications.
Standard Operating Procedures in the Lab
Every lab, whether it’s a university setting or an industrial facility, relies on Standard Operating Procedures (SOPs). These procedures ensure consistency and safety across different experiments. They act like a handbook guiding researchers through every step of their process, from sample preparation to waste disposal.
In developing SOPs, it’s critical to include precise details. For instance, if a procedure involves measuring liquid nitrogen, specifying the type of gloves to wear is crucial for safety. Moreover, these procedures are not set in stone; regular reviews help keep them relevant as new best practices emerge. Implementing effective SOPs fosters an environment where science thrives without unnecessary risks, thus streamlining the experimentation process.
Innovations in Experimental Techniques
In the realm of chemistry, innovation is a constant companion. The incorporation of advanced experimental techniques has allowed scientists to push the boundaries of what is possible. This part of the article will focus on three notable innovations that have dramatically changed the landscape of experimental chemistry.
Spectroscopy
Spectroscopy stands out due to its ability to provide deep insights into molecular structures and behaviors. By measuring light absorption, emission, or scattering, this technique allows chemists to deduce the functional groups present in organic compounds. The key characteristic of spectroscopy is its capacity to analyze substances without altering them. This non-destructive nature makes it incredibly valuable, especially in biomedical applications.
One unique feature of spectroscopy is its versatility. Techniques like nuclear magnetic resonance (NMR) and infrared (IR) spectroscopy serve distinct purposes but share the core goal of elucidating chemical structures. However, one must mention that the interpretation of spectra can sometimes be complex, requiring experience and expertise.
Chromatography
Chromatography has changed the game when it comes to separating mixtures. This technique makes it possible to isolate individual components from complex samples. The most notable characteristic of chromatography is its adaptability. Variations like gas chromatography (GC) and high-performance liquid chromatography (HPLC) cater to different needs within chemical research.
What truly sets chromatography apart is its precision. By optimizing parameters like temperature and mobile phase, chemists can refine separation processes to unprecedented levels of efficiency. A downside, however, could be the significant setup required for these methods, sometimes requiring specialized equipment and careful maintenance.
Electrochemical Methods
Finally, we delve into Electrochemical Methods. These techniques are essential for studying reaction mechanisms and quantifying concentrations of ionic substances. A defining characteristic of electrochemical methods is their ability to leverage chemical reactions to produce electrical signals. This property has led to widespread usage in sensors and environmental monitoring.
A unique feature of electrochemical methods is their real-time data collection. Unlike some traditional methods, these techniques can provide instantaneous feedback on changes in concentration or the onset of a reaction. However, they may face challenges with interference from other ions present in complex samples, which can affect results and require thorough calibration.
In summary, methodologies in chemistry are not merely frameworks; they serve as the very lifeblood of experimentation, ensuring that scientific inquiry remains rigorous, reliable, and revolutionary.
Safety and Ethical Practices in Chemical Experimentation
Safety and ethical considerations are paramount in the realm of chemistry experiments. These practices ensure not only the protection of individuals involved in the experimental processes but also uphold the integrity of scientific research as a whole. Adhering to strict safety protocols helps to prevent accidents and environmental hazards, while ethical practices promote responsible conduct within the field. This section delves into various critical components that define safe and ethical experimentation in chemistry.
Chemical Hazard Identification
Identifying hazardous chemicals is the first line of defense for any laboratory undertaking chemical experiments. Knowledge of these hazards enables researchers to implement appropriate risk management strategies before experiments are carried out. Many chemicals possess inherent dangers that can cause both acute and chronic health issues, including toxicity, flammability, or corrosiveness.
A thorough understanding of Material Safety Data Sheets (MSDS) is essential as they provide critical information regarding handling chemicals safely, including possible side effects and emergency procedures.
Some key points to consider in chemical hazard identification include:
- Labeling: Ensure that all chemicals are clearly labeled with their contents and associated hazards.
- Storage: Chemicals should be stored according to compatibility, avoiding dangerous reactions due to inappropriate placement.
- Training: Regular safety training sessions can help lab personnel remain aware of new hazards as they arise.
"Accident prevention in the lab is like locking the barn door after the horse is gone—you want to prevent problems before they happen."

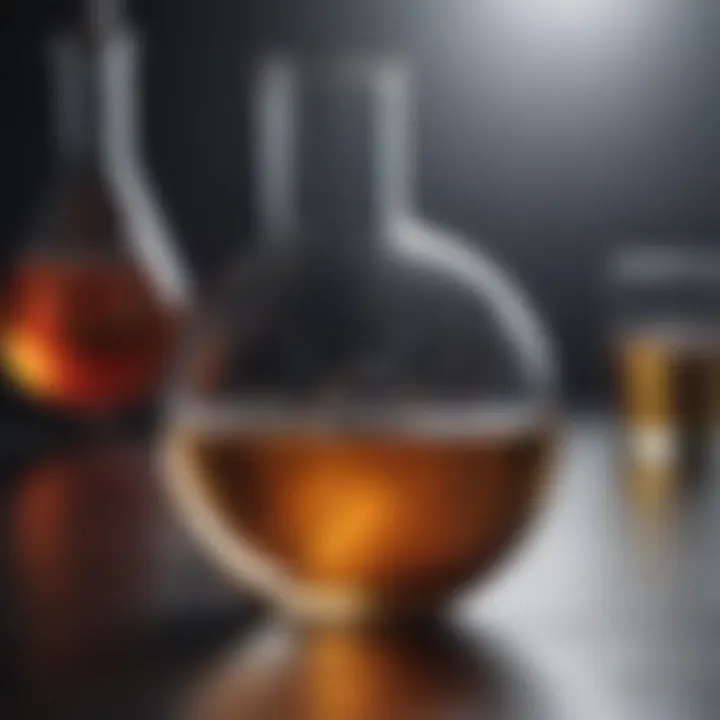
Personal Protective Equipment and Protocols
Personal Protective Equipment (PPE) serves as the body’s armor in chemical laboratories. Depending on the experimental nature, suitable PPE plays a critical role in shielding researchers from chemical exposure.
Common forms of PPE include:
- Gloves: Use appropriate gloves made from materials effective against specific chemical interactions.
- Goggles: Protective eyewear is crucial to safeguard against chemical splashes or flying debris.
- Lab Coats: Wearing lab coats prevents contaminated clothing and skin exposure during experiments.
In addition to the physical equipment, implementing protocols associated with PPE usage is equally important. These should encompass proper donning and doffing practices to avoid cross-contamination, regular inspections for wear and tear, and ensuring that all personnel understands how to select appropriate gear for their tasks.
Ethical Considerations in Chemical Research
Ethics in chemical research cannot be overlooked; the implications of experimental outcomes can extend beyond the lab, affecting communities and ecosystems. Researchers must be mindful of their responsibilities, acting with integrity in every step of experimentation.
Key ethical considerations include:
- Transparency: Researchers should document and share their methods and results openly to promote reproducibility and credibility within the scientific community.
- Accountability: Researchers must take responsibility for their findings and their implications, including potential health and environmental impacts.
- Respect for Living Organizations: When experiments involve biological materials, adherence to ethical guidelines regarding the treatment of these entities is crucial.
Balancing scientific inquiry with ethical standards ensures trust in chemical research and fosters a culture of respect among peers, institutions, and society at large.
By prioritizing safety and ethical practices, researchers can create an environment that respects both human life and the reputation of the scientific community as a whole.
Interdisciplinary Applications of Chemistry Experiments
The intersection of chemistry with other scientific disciplines adds layers to both understanding and application of chemical experiments. By examining how chemistry interfaces with fields like environmental science, medicine, and material science, we grasp the broader implications of our findings. Each discipline presents unique questions and challenges, creating a rich tapestry of inquiry that drives innovation. For students, researchers, and professionals alike, recognizing these crossroads deepens not only their comprehension of chemistry but also its societal relevance.
Chemistry in Environmental Science
Chemistry is at the forefront of tackling environmental issues, serving both as a diagnostic tool and a means for remediation. Through analysis of chemical compounds present in air, water, and soil, chemists can detect pollutants and assess their impact on ecosystems. For instance, the study of heavy metals like lead and mercury in water bodies illuminates how human activities can poison natural habitats.
Moreover, chemical experimentation plays a crucial role in understanding climate change. Researchers examine greenhouse gas emissions and their effects on global warming. Techniques like chromatography help separate and identify the various gases contributing to climate change, enabling targeted strategies for reduction. Sustainability initiatives also rely on chemistry to develop biodegradable materials and alternative energies, emphasizing a pressing need for interdisciplinary collaboration.
Role of Chemistry in Medicine
In the realm of medicine, chemistry is paramount. The development of pharmaceuticals hinges on chemical experiments that identify viable compounds capable of treating ailments. Understanding the pharmacokinetics and pharmacodynamics of these compounds involves intricate chemical methodologies. For instance, the development of vaccines against diseases like COVID-19 relied heavily on organic and medicinal chemistry to ensure efficacy and safety through rigorous testing phases.
Chemistry also underpins diagnostic methods, such as blood tests and imaging techniques. The way reagents interact in a laboratory setting can provide insights into a patient’s health status. For example, using spectroscopy to analyze blood can reveal concentrated levels of biomarkers indicative of diseases. Thus, the bond between chemistry and medicine illustrates an innovative yet practical synergy, leading to improved healthcare solutions.
Impact of Chemistry on Material Science
Material science, a field at the cutting-edge of technology, is another area enriched by chemistry experiments. The quest for new materials often involves investigating the properties and behaviors of various compounds. Consider the developments in nanotechnology, where chemists manipulate substances on a molecular scale to create materials with enhanced properties. These materials can exhibit unusual strength, lightweight characteristics, or conductivity—qualities that hold potential for applications in electronics, construction, and beyond.
Polymers offer a tangible example of chemistry’s impact on material science. The process of polymerization, explored through various chemical experiments, leads to innovations in everyday items, from packaging to medical devices. Furthermore, the ongoing research into smart materials, which can respond dynamically to environmental changes, highlights the interdisciplinary nature and collaborative spirit essential to advancing this field.
Chemistry serves as a backbone in the evolution of various technologies and methodologies in material science, driving exploration that holds promise for future advancements.
"The fusion of chemistry with other disciplines is not just beneficial; it’s essential for progress in tackling the world's pressing issues."
The interdisciplinary applications of chemistry experiments exemplify the intricate web of connections within the sciences, inspiring future investigations that seek to enhance knowledge and address real-world problems.
Data Analysis and Interpretation in Chemistry Experiments
Data analysis and interpretation plays a vital role in the realm of chemistry experiments. While conducting experiments can yield fascinating results, the real challenge often lies in deciphering those results and determining what they actually mean in a broader context. The way that data is analyzed not only affects the conclusions one draws but also impacts the reproducibility and validity of the findings. In this section, we will dissect the significance of data analysis, the methods used, and how the interpretation shapes future scientific inquiries.
Statistical Methods in Chemical Research
Statistical methods are the backbone of data analysis in chemical research. They allow chemists to draw meaningful conclusions from often complex datasets. Some key statistical methods employed are:
- Descriptive Statistics: This includes measures like mean, median, mode, and standard deviation, which summarize data points effectively.
- Inferential Statistics: Techniques such as hypothesis testing, confidence intervals, and regression analyses help researchers make inferences or generalizations about a population based on sample data.
- ANOVA (Analysis of Variance): This method distinguishes between multiple groups to see if at least one group mean is different from others, which is crucial in experiments with multiple variables.
- Chi-Squared Tests: This technique assesses how likely it is that an observed distribution is due to chance, especially important in categorical data analysis.
Each of these methods provides a unique lens through which data can be viewed and interpreted. Keep in mind that misapplication of these methods can lead to skewed results, which can have far-reaching consequences.
Interpreting Experimental Results
Interpreting results from chemistry experiments requires a mix of analytical skills and contextual knowledge. Once data has been analyzed statistically, a chemist must consider several factors:
- Contextual Relevance: Results must be interpreted in the context of existing literature. How do the findings compare with previous studies? This can offer insights into the reliability and novelty of the current research.
- Anomalies and Outliers: Identifying any data points that don't seem to fit is crucial. Anomalies can suggest experimental errors, but they can also point toward unexpected trends or new phenomena.
- Theoretical Framework: Often, results need to be assessed against theoretical predictions. If the data diverges significantly from what theory suggests, it might prompt a reevaluation of the underlying assumptions.
- Practical Implications: Finally, the practical applications of the results should be examined. How can the findings be utilized in real-world settings? Understanding this can drive future research and innovation in fields like material science and pharmaceuticals.
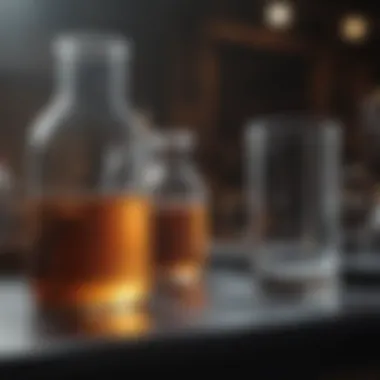
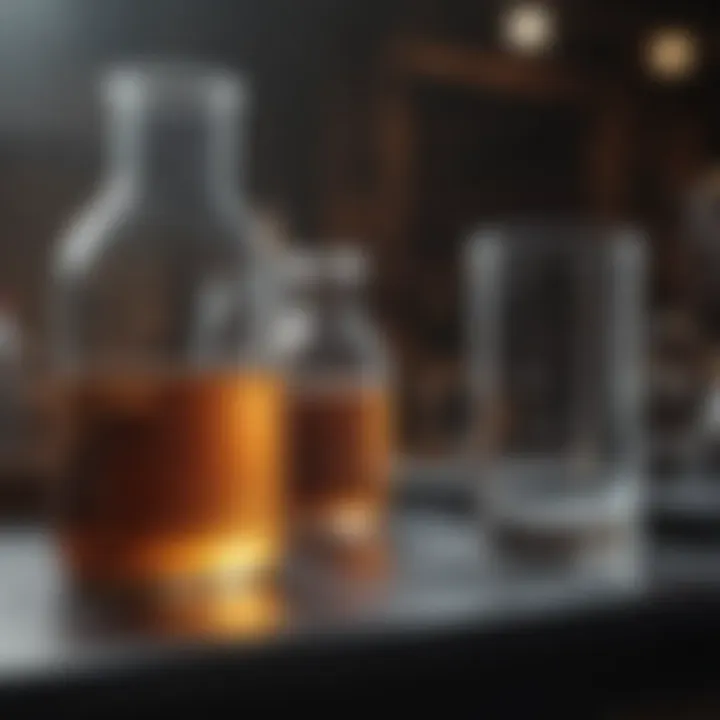
Challenges and Limitations in Chemistry Experiments
Understanding the challenges and limitations in chemistry experiments is pivotal for researchers and educators alike. While experiments are fundamental to uncovering the intricacies of chemical phenomena, they often come laden with pitfalls that can skew results and hinder scientific progress. Addressing these challenges not only sharpens experimental design but also fosters a culture of critical thinking in both educational institutions and research laboratories.
In the realm of chemistry, experiments serve as a bridge connecting theoretical concepts with practical application. However, it's crucial to recognize that not all experiments go off without a hitch. Acknowledging the potential for errors and limitations is essential for evaluating the validity of experimental data. This section will delve into common errors encountered during experimentation and the inherent limitations of current methodologies used in the field.
Common Experimental Errors
Every chemist has likely heard the phrase, "Mistakes are the portals of discovery." This sentiment rings true, yet that doesn’t lessen the frustration when they do occur. Common experimental errors can arise from a multitude of sources, including:
- Human Error: Simple mistakes in measurements or calculations can lead to significant discrepancies in results. For instance, misreading a graduated cylinder can throw off the concentration of a solution, altering the outcome of the entire experiment.
- Contamination: One of the sneakiest culprits, contamination can alter the composition of chemical substances, leading to erroneous conclusions. It can occur from cross-contamination between samples or even improper cleaning of glassware.
- Equipment Calibration: If instruments are not calibrated properly, the data they provide can be misleading. For example, an improperly calibrated thermometer can present temperature readings that are off, affecting reactions that are temperature sensitive.
- Environmental Factors: External factors like temperature fluctuations, humidity, and even vibrations can affect experimental outcomes. These are often outside the researcher’s control but must be acknowledged when analyzing results.
By understanding these common pitfalls, chemists can adopt preventative measures to enhance the reliability of their findings.
Limitations of Current Methodologies
Despite the advancements in experimental techniques, certain limitations remain in methodologies employed in chemistry labs. These constraints can affect the scope and reliability of experiments.
- Scope of Application: Many traditional experimental methods are designed for specific conditions and may not perform well when adapted to different environments or scales. For instance, methods developed for small-scale reactions might not yield the same accuracy when applied to industrial-scale processes.
- Reproducibility Issues: Studies have shown that some experiments struggle with reproducibility due to slight variations in techniques, sample preparation, or even the batch variability of chemical reagents. This hiccup poses significant barriers to validating research findings.
- Resource Intensity: Some analytical techniques require extensive resources, such as time and manpower, which can limit their feasibility, especially in educational settings. Underfunded labs often can’t afford top-tier equipment, which may skew the breadth and depth of experiments conducted.
- Technological Constraints: While some innovative technologies are on the rise, others remain outdated. Techniques such as older spectroscopic methods may not provide the speed and sensitivity needed for contemporary chemical analyses, leading to a reliance on less effective methods.
In summary, being conscious of these limitations sets the stage for refining methodologies, promoting more rigorous scientific inquiry, and fostering a mindset that embraces adaptability and innovation in experimental chemistry.
Future Directions in Chemistry Experiments
The field of chemistry is ever-evolving, with each discovery carving new paths for further exploration. Future directions in chemistry experiments promise not just to refine our current understanding but to revolutionize how we approach scientific inquiries. The significance of these future trends cannot be overstated.
Emerging Technologies in Chemical Research
The future of chemistry is intricately linked with technological advancement. Tools and techniques being developed today are set to transform the landscape of chemical research. For instance, the rise of microfluidics enables chemists to conduct experiments on a miniature scale, allowing for faster and more efficient reactions. This innovation reduces the amount of reagents necessary, yielding cost-effective research methods.
In addition, high-throughput screening is gaining traction. Rather than testing one variable at a time, this technique allows scientists to evaluate many compounds simultaneously. This capability is especially crucial in drug discovery, where researchers aim to identify potential therapeutic compounds swiftly. Let’s break down some exciting emerging technologies:
- Nanotechnology: Harnessing the properties of materials at the nanoscale, this field enhances precision in material science and biological applications.
- Green Chemistry: Focused on sustainability, green chemistry emphasizes reducing hazardous substances and waste, providing a vital framework for future experimentation.
- Advanced Spectroscopic Techniques: Improvements in imaging and analysis methods enable molecular characterization with unmatched accuracy.
"The marriage of technology and chemistry paves the way for unparalleled discoveries that can tackle society’s pressing issues."
These technologies underscore a trend towards more intricate and multifaceted chemistry experiments, enabling researchers to address complex questions previously deemed insurmountable.
Integrating Artificial Intelligence in Experimental Chemistry
Artificial intelligence, once a concept relegated to science fiction, now has tangible applications in chemical research. The integration of AI enhances data analysis and interpretation, allowing for faster and more insightful conclusions.
Consider a scenario where vast datasets from spectroscopy and chromatography are analyzed. Manually sifting through this data can be tedious and prone to human error. AI algorithms can identify patterns and anomalies far more quickly and accurately. Advantages of using AI in experimentation include:
- Predictive Modeling: AI can be trained to predict outcomes of reactions before they are even conducted, saving resources and time.
- Optimization of Experiment Conditions: By analyzing past experiments, AI can suggest optimal conditions for future studies, accommodating numerous variables.
- Automated Synthesis: Robotic systems powered by AI can autonomously conduct experiments. This capability not only increases throughput but minimizes human error.
However, as with any tool, the integration of AI must be approached with care. Researchers need to ensure these systems complement their work rather than distort the pure essence of scientific inquiry. Embracing AI's potential while remaining vigilant to its limitations will define the next chapter of chemical experimentation.
The future undoubtedly holds exciting opportunities for chemistry experiments, driven by emerging technologies and artificial intelligence. These advancements lead us not just towards enhanced efficiency but also towards a deeper understanding of the chemical universe, fulfilling the age-old quest for knowledge.
Finale
The conclusion of this article encapsulates the enduring importance of chemistry experiments within scientific inquiry. Throughout the various sections, we have dissected how experiments act as the backbone of chemical understanding, shedding light on both fundamental principles and advanced methodologies. Each piece of information reiterates the complexity involved in conducting chemical research, emphasizing that every experiment contributes uniquely to our larger comprehension of the subject.
The Ongoing Relevance of Chemistry Experiments
Chemistry experiments are not just mere academic exercises; they hold a pivotal role in shaping myriad fields from healthcare to environmental science. The ongoing relevance can be observed in several key factors:
- Continuous Innovation: The landscape of chemistry is ever-changing. With the rise of new technologies, methods like spectroscopy and chromatography are not only enhancing our understanding but enabling faster, more accurate results.
- Interdisciplinary Applications: The knowledge derived from chemistry experiments feeds into various domains such as biochemistry, materials science, and energy solutions, making it indispensable. For instance, advancements in materials chemistry impact everything from electronics to renewable energy.
- Real-World Solutions: As we face global challenges like climate change and health crises, the importance of empirical data through experiments becomes clear. They serve as a foundation for developing solutions that are not only effective but also sustainable.
"Scientific progress is built on the bedrock of rigorous experimentation, where each finding could lead to nerve-wracking breakthroughs or cautionary tales of failure."
The ongoing efforts in refining methodologies and the integration of technological advancements make it evident that chemistry experiments are vital. Researchers and educators must foster a culture that prioritizes experimental skill development, ensuring that future generations are equipped to tackle the upcoming challenges head-on.
In summary, the relevance of chemistry experiments lies not only in their historical significance but also in their ability to advance human knowledge and contribute to societal welfare. They are essential tools for understanding our world and crafting solutions for a better future.