Exploring the Frontiers of Synthetic Biology
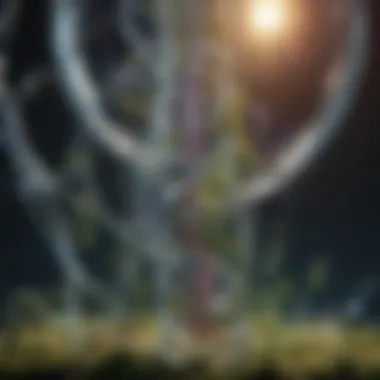
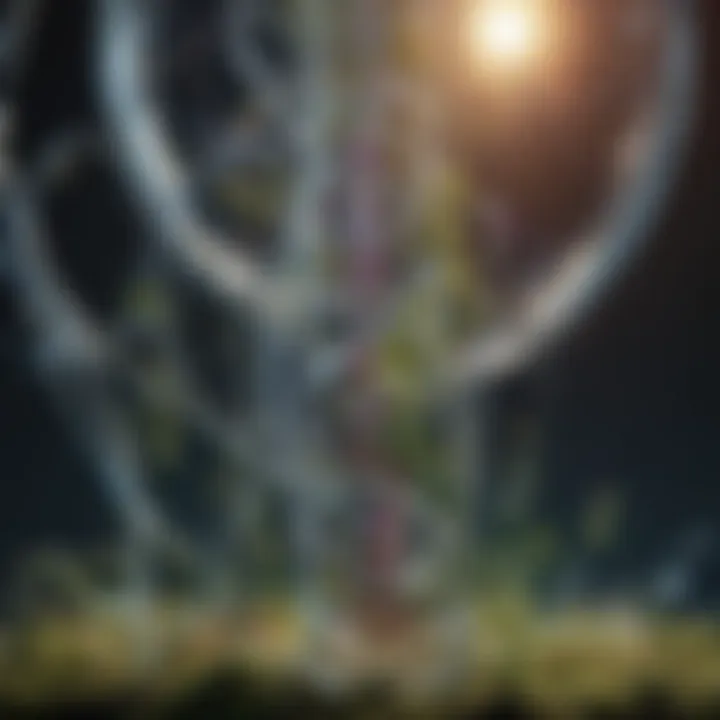
Intro
Synthetic biology is a rapidly evolving multidisciplinary field that fuses biology with engineering principles. Its primary goal is to design and construct new biological parts and systems. By utilizing the tools of molecular biology and genetic engineering, researchers can manipulate the genetic codes of organisms, creating new functionalities. This offers great possibilities across various sectors, including medicine, agriculture, and environmental management.
Synthetic biology encourages innovation by allowing scientists to reprogram organisms or create entirely new ones. These advancements promise to enhance our understanding of biological systems while yielding practical solutions to pressing global challenges.
As synthetic biology progresses, it brings to the fore essential ethical considerations, calling into question the moral implications of altering life forms. Understanding its underpinnings is crucial for both informed decision-making and future research initiatives in this ever-expanding field.
Key Concepts
Definition of Primary Terms
Synthetic biology is often defined as an engineering discipline that involves the creative redesign of existing biological systems or the construction of new ones. It combines techniques from various scientific fields including genetics, biotechnology, and computer science to create organisms with specified functions. Key terms include:
- Biobricks: Standardized sequences of DNA that can be assembled in various combinations.
- Gene Editing: Techniques such as CRISPR-Cas9 that allow precise modifications to DNA sequences.
- Metabolic Engineering: Adjusting the metabolic pathways of organisms to optimize the production of certain substances.
Related Concepts and Theories
Several theories underscore synthetic biology’s foundation. Among these, the Central Dogma of Molecular Biology, which describes the flow of genetic information from DNA to RNA to protein, is pivotal. Additional concepts include systems biology, which seeks to understand complex biological systems through an integrated approach, and bioinformatics, which uses computational methods to analyze biological data. Together, these frameworks provide a comprehensive understanding of how synthetic biology operates.
"Synthetic biology represents a revolution in the biological sciences, enabling us to design life and address some of the world's most pressing needs."
Future Directions
Gaps Identified in Current Research
Despite its advancements, synthetic biology faces significant gaps, particularly in regulatory and safety assessments. Current research often lacks comprehensive frameworks for addressing the unexpected behaviors of engineered organisms in ecological settings. There are also critical areas needing exploration, like the long-term effects of synthetic organisms on biodiversity and ecosystem stability.
Suggestions for Further Studies
To further advance the field, future studies should focus on several key areas:
- Developing robust regulatory frameworks to assess risks associated with synthetic organisms.
- Investigating the socioeconomic impacts of synthetic biology applications.
- Enhancing interdisciplinary collaboration to integrate insights from diverse scientific domains.
In summary, synthetic biology is a dynamic field that melds biology with engineering principles, paving the way for innovative solutions. While it holds remarkable potential, it also necessitates thoughtful consideration of its ethical implications and societal impacts.
Prelims to Synthetic Biology
Synthetic biology emerges as a transformative field that bridges the gap between biological sciences and engineering. The importance of this discipline cannot be understated, particularly in its ability to innovate and design biological systems that can solve pressing global challenges. From medical advancements to environmental sustainability, synthetic biology offers an array of solutions that leverage the inherent capabilities of living organisms.
Its relevance is especially significant in a world where traditional methods may be insufficient to tackle issues like disease outbreaks, food insecurity, and climate change. By employing synthetic biology, we can engineer microorganisms to produce biofuels, design cells that target cancer cells specifically, or even create plant varieties with enhanced nutritional profiles. As society grapples with these complex problems, the innovative approaches facilitated by synthetic biology will play a crucial role in enhancing human well-being.
Additionally, it spurs discussions surrounding ethical considerations and regulatory frameworks. As the capabilities of synthetic biology expand, so too does the need to ensure the responsible application of these technologies.
Here's a structured overview of important elements in synthetic biology:
- Builidng blocks of life: It involves the digital representation of biological constructs.
- Engineering principles: It encompasses the design and assembly of genetic circuits.
- Applications: These range widely, impacting medicine, agriculture, and environmental science.
By exploring these elements, this article endeavors to provide a comprehensive overview of synthetic biology, paving the way for an informed dialogue on its future and implications.
Definition and Scope
Synthetic biology can be broadly defined as the design and construction of new biological parts or systems. It also includes the re-engineering of existing natural biological systems for useful purposes. The scope of synthetic biology extends across various fields, blending biology, engineering, and computational sciences to achieve its goals.
One major aspect of this discipline is the creation of standardized biological parts. These parts can be assembled in various configurations to create new functionalities. BioBricks are a popular example, serving as standardized units that can be combined for diverse applications. This modularity allows for innovations that were once deemed impossible, thus expanding the horizons of what biology can achieve.
Historical Context
The origins of synthetic biology can be traced back to earlier developments in genetics and molecular biology. The discovery of DNA's structure in the 1950s laid the foundation for genetic manipulation. However, the term "synthetic biology" was not widely used until the early 2000s, when scientists sought to formulate standardized approaches to genetic engineering.
The first synthetic cell, created by the J. Craig Venter Institute in 2010, marked a significant milestone in this field. This achievement highlighted the potential of synthetic biology to not only edit existing organisms but to create entirely new life forms from scratch.
As the field evolved, it integrated tools and techniques from various disciplines, enabling a more holistic approach to biological engineering. This interdisciplinary nature allows for greater collaboration among researchers, paving the way for significant advancements in understanding and manipulating biological systems.
The historical journey of synthetic biology illustrates its remarkable evolution from basic genetic manipulation to a sophisticated field capable of addressing some of humanity’s most urgent challenges.
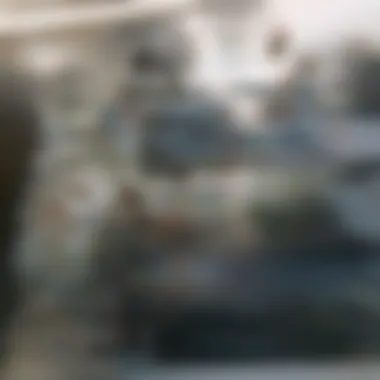
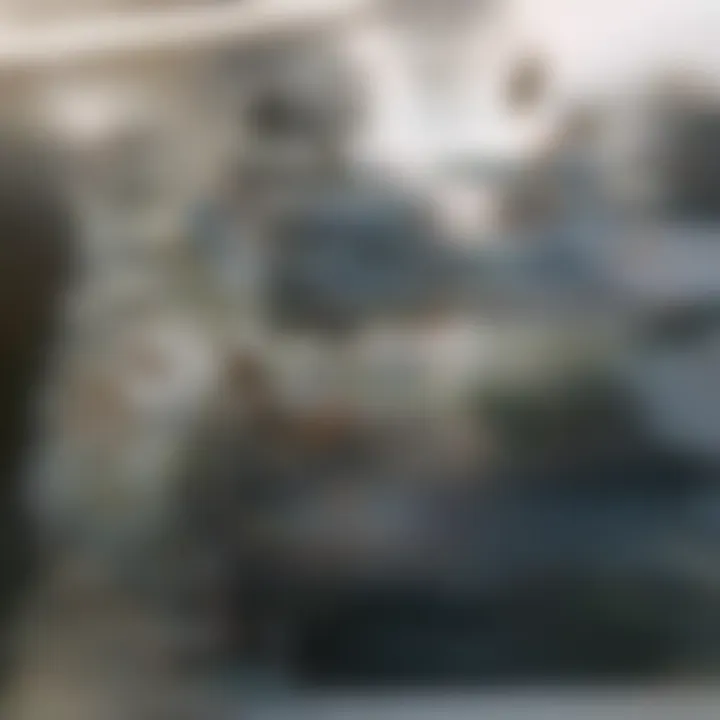
Fundamental Concepts in Synthetic Biology
The foundation of synthetic biology is built on fundamental concepts that interlink biological science with engineering principles. Understanding these concepts is crucial for advancing the field. They include genetic engineering techniques, modular design principles, and a series of biological parts and systems. Each aspect plays a significant role in how synthetic biology can create, modify, and optimize biological systems for various applications.
Genetic Engineering Techniques
Genetic engineering techniques are central to synthetic biology, allowing scientists to manipulate genetic material. The most recognized methods include CRISPR technology, gene cloning, and gene synthesis.
CRISPR Technology
CRISPR technology has revolutionized genetic engineering due to its precision and efficiency. It allows for specific alterations in the genome by utilizing guide RNAs that direct the Cas9 enzyme to desired DNA locations. This specificity is what makes CRISPR a beneficial choice for synthetic biology. Its ability to edit genes in living organisms has massive implications for medical therapies, agriculture, and more. However, ethical concerns regarding off-target effects remain a disadvantage in its application.
Gene Cloning
Gene cloning enables the replication of specific genes to study their functions or produce proteins. This method has been integral in creating organisms that express specific traits. Gene cloning offers simplicity and efficiency, making it a popular choice in research and development. The downside is that it can be time-consuming and may not always yield the desired clones, leading to potential inefficiencies in some cases.
Gene Synthesis
Gene synthesis involves constructing genes from scratch using chemical methods. This technique allows researchers to create entirely new genes, which is essential for designing organisms with specific functionalities. Gene synthesis enables fast prototyping of genetic constructs, which is very beneficial for innovation. A potential limitation is the cost associated with synthesizing longer or more complex sequences, which can hinder widespread applications.
Modular Design Principles
Modular design principles are critical for simplifying the construction of complex biological systems. By breaking down biological components into interchangeable parts, researchers can design and build new functionalities with ease. This approach enables innovation and reduces the time needed to develop new synthetic organisms or pathways. Using modular designs can also lead to more predictable outcomes when creating biological systems, which is essential for reliable applications.
Biological Parts and Systems
Understanding biological parts and systems is fundamental in building synthetic biology applications. This includes components like BioBricks, gene circuits, and metabolic pathways.
BioBricks
BioBricks are standardized sequences of DNA that can be easily combined with other sequences. They promote modularity and enable the construction of complex genetic systems efficiently. The key feature of BioBricks is their standardization, which simplifies the design process and enhances collaboration among researchers. The limitation of BioBricks lies in their reliance on existing parts; creating new BioBricks can require additional effort and experimentation.
Gene Circuits
Gene circuits are designed constructs that behave like electronic circuits but within biological systems. They can regulate gene expression levels in response to environmental stimuli. Their key characteristic is programmability, allowing researchers to build specific functions into cells. Though there are advantages, such as versatility in applications, gene circuits often encounter challenges like variability in cellular responses among different host organisms.
Metabolic Pathways
Metabolic pathways involve a series of chemical reactions occurring within a cell. Understanding and manipulating these pathways can lead to enhanced production of desired substances, like biofuels or pharmaceuticals. Their key characteristic is the interconnected nature of reactions, making pathway engineering a complex yet rewarding area of study. However, engineers must consider cellular resource allocation and potential bottlenecks, as these can limit pathway efficiency.
Technological Advancements in Synthetic Biology
The field of synthetic biology is rapidly evolving, characterized by a series of technological advancements that are reshaping its landscape. These advancements play a crucial role in boosting the potential applications of synthetic biology across various sectors, including medicine, agriculture, and environmental science. By leveraging cutting-edge tools and techniques, researchers can design and modify biological systems with unprecedented precision, making it vital to explore these developments thoroughly.
Synthetic Genomes
Synthetic genomes are a groundbreaking innovation in synthetic biology. They refer to the artificially constructed DNA sequences that can replicate the functions of natural genomes. Creating synthetic genomes allows scientists to study genetics in greater detail, enhancing the understanding of genetic codes and their roles in cellular functions. This innovation is particularly important for producing organisms with desirable traits, such as increased resistance to diseases or enhanced yield levels in crops. The ability to construct synthetic genomes has opened new avenues for creating living organisms tailored for specific needs.
Cell-Free Systems
Cell-free systems streamline the process of synthetic biology by enabling biological reactions to occur outside living cells. Such systems reduce variability seen in traditional cell-based experiments. Researchers can manipulate the components more easily, leading to higher efficiency in metabolic reactions. The use of cell-free systems facilitates rapid prototyping of biological materials, enhancing the pace of research and development in areas like biopharmaceuticals and biosensors. Cell-free biology is an emerging area that allows more flexible and controlled experimentation.
Computational Tools
Modeling Biological Systems
Modeling biological systems provides a framework to understand complex biological interactions. This computational approach allows researchers to simulate cellular processes, predict outcomes, and optimize conditions for desired results. One key characteristic of modeling is its ability to integrate vast amounts of biological data, which enhances accuracy in predictions. This method is beneficial in synthetic biology, as it helps anticipate how engineered organisms will behave in real-world conditions. Unique features of modeling include its capacity to simulate changes over time, which is crucial for studies on metabolic pathways and gene regulation. However, limitations exist in accurately representing every variable in a biological system, leading to potential discrepancies between model predictions and actual outcomes.
Bioinformatics Applications
Bioinformatics applications serve as a vital component in the analysis and interpretation of biological data. These tools process vast datasets generated from genomic sequencing, enabling researchers to identify genes of interest and predict their functions. A key characteristic of bioinformatics is the ability to transform raw data into actionable insights, which supports the development of new synthetic biology applications. Unique features include algorithms that assist in aligning DNA sequences and predicting structural compositions of proteins. This field has advantages like increased efficiency in data processing, but it also faces challenges in integrating diverse datasets from multiple sources, which can complicate analyses.
"Technological advancements in synthetic biology are not merely tools but gateways to redefining biological constructs and ecosystems."
In summary, the advancements in synthetic genomes, cell-free systems, and computational tools are pivotal in propelling the field of synthetic biology forward. These innovations not only enhance understanding of biological processes but also lead to practical applications that can significantly influence medicine, agriculture, and environmental remediation.
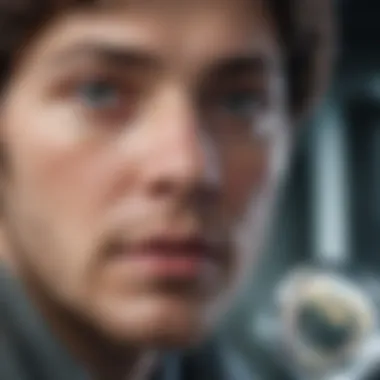
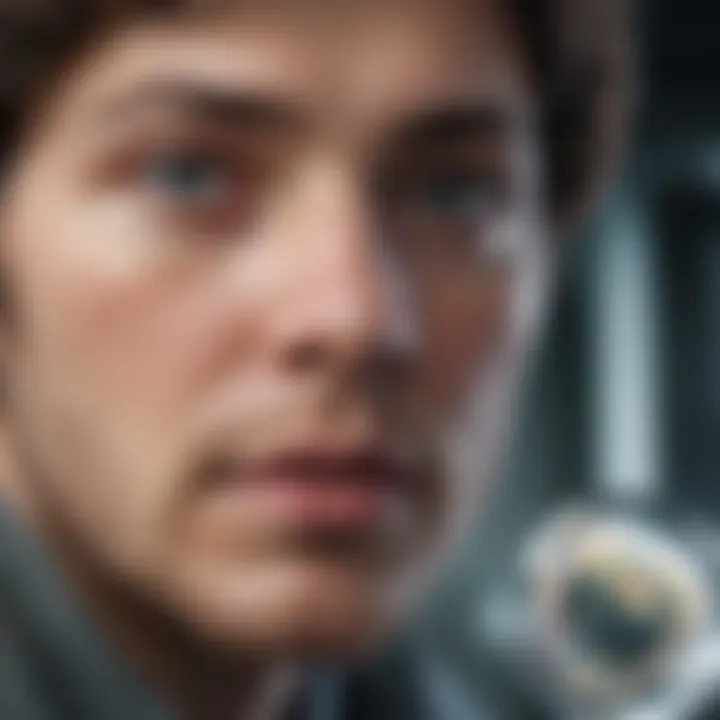
Applications of Synthetic Biology
The applications of synthetic biology are vast and transformative. They span various fields including medicine, agriculture, and environmental science. The importance of this topic lies in its potential to address some of the most pressing challenges faced by humanity today. As the world grapples with issues such as disease, food insecurity, and climate change, synthetic biology emerges as a beacon of innovation. Understanding these applications illuminates the relevance of synthetic biology in contemporary society.
Medical Innovations
Gene Therapy
Gene therapy represents a groundbreaking approach to treating genetic disorders by introducing or altering genes within a patient’s cells. This technique allows for the correction of mutations at their source. The key characteristic of gene therapy is its focused nature, specifically targeting the underlying cause of a disease rather than just alleviating symptoms. This makes it a desirable choice in the realm of medical innovations.
A unique feature of gene therapy is its ability to provide long-lasting therapeutic effects from a single intervention. Advantages include the potential for permanent correction of conditions like cystic fibrosis or muscular dystrophy. However, challenges exist, such as the risk of immune responses against the therapeutic agents and the difficulty in delivering the gene modifications effectively to the right cells.
Vaccine Development
Vaccine development has taken significant strides through synthetic biology. This aspect contributes profoundly to public health by enhancing immune responses and creating targeted vaccines. The rapid adaptability of synthetic biology methodologies makes it a cornerstone of efficient vaccine development for emerging infectious diseases, such as the COVID-19 pandemic.
The key characteristic of synthetic vaccines is their ability to be quickly designed and manufactured. For example, mRNA vaccines utilize synthetic constructs to instruct cells to produce antigens that trigger an immune response. This innovation offers advantages such as improved safety profiles and the possibility of less reliance on live pathogens. Nonetheless, challenges persist in terms of ensuring long-term efficacy and dealing with public vaccine hesitance.
Agricultural Enhancements
Genetically Modified Organisms
Genetically modified organisms (GMOs) are organisms whose DNA has been altered using genetic engineering techniques. They play a pivotal role in reshaping agricultural productivity. The key characteristic of GMOs is their ability to exhibit desirable traits, such as pest resistance or enhanced nutritional content. This makes them an advantageous choice for increasing food security and addressing malnutrition.
A unique feature of GMOs is their potential to reduce reliance on chemical pesticides and fertilizers, contributing to more sustainable farming practices. The advantages of using GMOs include higher crop yields and reduced environmental impact. However, there are concerns about biodiversity loss and the long-term effects of consuming GMOs, leading to ongoing debates about their safety.
Sustainable Practices
Sustainable practices in agriculture involve methods that meet current food needs without compromising the future. They are essential for minimizing ecological footprints. The key characteristic of sustainable farming is its holistic approach, integrating environmental health, economic profitability, and social equity. This practice stands out due to its aim of achieving balance in agricultural ecosystems.
A unique feature of sustainable practices is the promotion of biodiversity through crop rotation and organic farming. The advantages of these methods include improved soil health and resilience against pests. Nevertheless, challenges persist, such as the need for rigorous education and support systems for farmers transitioning to sustainable methods.
Environmental Applications
Bioremediation
Bioremediation is the process of using living organisms to remove or neutralize contaminants from the environment. This application is critical in addressing pollution in soil and water. The key characteristic of bioremediation is its eco-friendly approach, harnessing natural biological processes to restore ecosystems.
A unique feature is the use of microorganisms, which can break down pollutants, making them advantageous for cleaning up hazardous waste sites. While bioremediation offers benefits such as cost-effectiveness and minimal disturbance to the environment, challenges remain, including the need for extensive monitoring and sometimes slow degradation rates of pollutants.
Carbon Capture Technologies
Carbon capture technologies aim to capture carbon dioxide emissions from various sources to mitigate climate change. This area represents a significant advance in addressing global warming. The key characteristic of these technologies is their ability to trap carbon before it enters the atmosphere, making them a valuable tool in reducing greenhouse gas emissions.
A unique feature of carbon capture is its versatility; it can be applied in various settings, including power plants and industrial sites. Advantages include the potential to significantly lower overall emissions. However, disadvantages exist, such as the high costs of implementation and energy requirements, necessitating ongoing research to enhance efficiency and scalability.
"Synthetic biology not only enhances our understanding of biological systems but also provides innovative solutions to some of today's critical challenges."
In summary, the applications of synthetic biology present a multitude of opportunities across diverse fields. These innovations not only address immediate concerns but also lay the groundwork for sustainable solutions in the future.
Ethical Considerations in Synthetic Biology
Understanding ethics in synthetic biology is crucial. This field intertwines life sciences with engineering. Thus, ethical issues extend across multiple domains. The implications of synthetic biology are increasingly profound as experiments push boundaries. Here, we explore two main areas: biosafety and biosecurity, and societal impacts.
Biosafety and Biosecurity
Biosafety refers to the measures taken to ensure that synthetic biology practices do not harm human health or the environment. This involves strict guidelines and protocols. Each step in synthetic biology must consider potential unintended consequences. Effective biosafety measures are crucial for building trust with the public.
Biosecurity involves preventing the misuse of synthetic biology. It aims to protect against malicious applications, like bioterrorism. Strong biosecurity frameworks encourage responsible research and innovation. Implementing such strategies helps safeguard not just physical entities but also ethical standards.
To maintain public trust, biosafety and biosecurity must be prioritized in all synthetic biology endeavors.
Societal Impacts and Public Perception
Public perception plays a vital role in shaping the direction of synthetic biology. People often have fears or misconceptions. Understanding these factors is essential for the further development of the field.
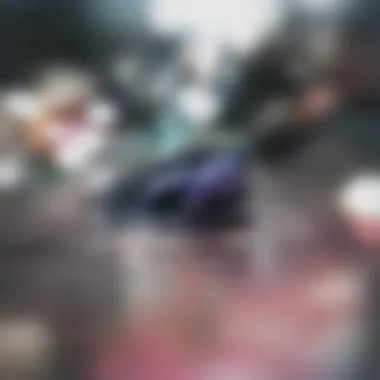
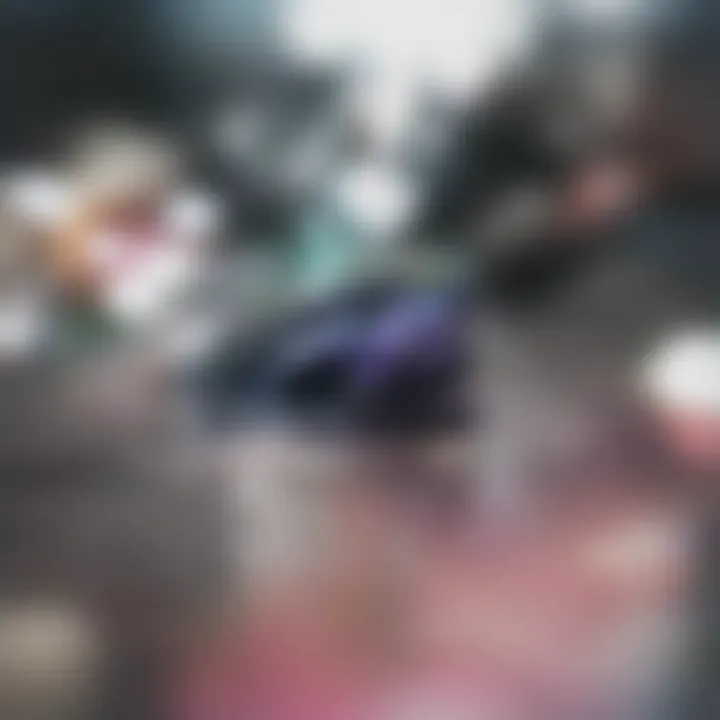
Risk Assessment
Risk assessment is the process of identifying and analyzing potential hazards. This aspect evaluates the likelihood and consequence of adverse effects from synthetic biology practices. Thorough risk assessments can help to mitigate public concerns. They provide a structured way to evaluate potential risks vs benefits.
A key characteristic of risk assessments is their systematic approach. This makes risk assessment a popular choice for addressing ethical considerations. However, limitations exist. Complex biological systems can be unpredictable, making risk predictions challenging.
Public Engagement
Public engagement involves meaningfully involving stakeholders in discussions about synthetic biology. It is fundamental for fostering understanding and acceptance. A crucial feature of public engagement is that it creates transparency. Transparent dialogue can address societal concerns head-on, giving voice to various opinions and fears.
The advantages of engaging the public include improved trust and informed decision-making. However, one unique challenge in public engagement is managing diverse perspectives. Not all groups will have the same values or concerns. This complexity must be carefully navigated to build productive conversations.
Ultimately, ethical considerations in synthetic biology require a balanced approach. Both biosafety and biosecurity, along with societal dialogue, contribute to an ethical framework. This not only ensures acceptance but also encourages responsible advancement.
Regulatory Frameworks for Synthetic Biology
Regulatory frameworks for synthetic biology are vital for ensuring the safe and responsible application of this innovative science. As synthetic biology advances rapidly, establishing clear guidelines and regulations can mitigate risks associated with genetic modifications, biosafety concerns, and ethical dilemmas. Increasing interaction between biology and engineering necessitates efficient oversight to protect public health, biodiversity, and environmental sustainability.
Importance of Regulatory Frameworks
- Safety: The key role of regulation is to prevent unintended consequences from synthetic biological experiments. A robust framework ensures that experiments do not pose risks to human health or the ecosystem.
- Public Trust: Transparency in regulation fosters public confidence. When people see that science operates under responsible oversight, they are more likely to accept advancements in synthetic biology.
- Innovation Facilitation: A clear regulatory environment can promote innovation by providing guidelines that researchers need to follow. This leads to efficient and timely breakthroughs.
- International Cooperation: A common regulatory framework supports international partnerships and exchanges of technologies, aligning efforts on a global scale.
International Guidelines
International guidelines serve as foundational structures that countries can reference when they create their own regulations. Organizations such as the World Health Organization (WHO) and the Organization for Economic Co-operation and Development (OECD) provide frameworks aimed at harmonizing practices across nations.
Key components of International Guidelines:
- Biosafety Assessments: Evaluate the risks associated with intentional releases of genetically modified organisms.
- Risk Management Strategies: Encourage practices that minimize risks in lab and field settings.
- Ethical Standards: Focus on ethical considerations related to freedom, privacy, and potential misuse of technology.
National Legislation
National legislation plays a critical role in defining how synthetic biology is conducted within each country. Regulatory bodies must adapt laws to reflect the evolving nature of synthetic biology technologies.
Examples of National Legislation:
- The U.S. Coordinated Framework for Regulation of Biotechnology: Integrates the work of various federal agencies to regulate biotechnology and synthetic biology without imposing excessive burdens.
- European Union’s Genetically Modified Organisms (GMO) Directive: Establishes a stringent framework for the assessment and monitoring of GMOs, reflecting the EU's precautionary approach.
- Biosafety Regulations in Brazil: Offers guidelines on the assessment of genetically modified products, promoting sustainable agriculture while ensuring safety.
Adhering to regulatory requirements ensures that the innovations in synthetic biology align with societal values and safety standards.
The establishment of regulatory frameworks both internationally and nationally is essential for the continuous advancement of synthetic biology. As societal needs and scientific capabilities evolve, ongoing discussions about regulations will help navigate the complexities associated with these frontier technologies.
Future Directions in Synthetic Biology
The future directions in synthetic biology signify a pivotal juncture for the discipline. The evolving landscape shows not only technological and scientific growth but also emerging societal implications that require keen examination. Innovations being developed now may lead to significant breakthroughs in various fields, with the potential to change healthcare, food production, and environmental conservation. A comprehensive understanding of future directions highlights the importance of staying ahead in this rapidly progressing field while addressing ethical dilemmas and societal readiness.
Innovations on the Horizon
Innovations in synthetic biology are unfolding at an unprecedented pace. New techniques, such as advanced gene editing and novel metabolic engineering, are becoming essential for the development of engineered organisms. One key area is the customization of microbial strains for specific biosynthetic pathways. This allows for more efficient production of biofuels, pharmaceuticals, and bioplastics. Also, researchers are exploring synthetic organisms with bespoke functionalities tailored to unique applications, such as waste breakdown and pollution reduction. The integration of artificial intelligence into synthetic biology could further refine experimental designs and prediction models, making the design of living systems more streamlined and effective.
"The growth of AI and machine learning offers unprecedented insights into biological data, revealing patterns that may lead to practical applications."
Collaborative Research Initiatives
Collaborative research initiatives are crucial for advancing synthetic biology. These initiatives often bring together interdisciplinary teams comprising biologists, engineers, and data scientists. For example, projects like the iGEM competition foster international collaboration, encouraging students to develop synthetic biology solutions for real-world challenges. Such cooperative frameworks can pool resources and expertise, streamline innovation processes, and enhance knowledge sharing. Partnerships across institutions and industries also enable large-scale projects that target global problems, such as climate crisis and energy sustainability. It is essential to engage in open collaboration to drive the field further and address pressing issues collectively.
Interdisciplinary Approaches
Interdisciplinary approaches in synthetic biology are imperative for its advancement. Integration with computer science enhances bioinformatics, which is vital for analyzing complex biological data. Additionally, the combination of synthetic biology with materials science can lead to the development of biologically compatible and sustainable materials. Collaborating with social scientists is also essential to understand the societal implications of synthetic biology innovations. This comprehensive approach not only promotes enhanced scientific discovery but also ensures that novel solutions are accepted and integrated into society responsibly. Engaging diverse fields helps to address ethical concerns, regulatory requirements, and public perception, leading to a more rounded and informed discourse around synthetic biology's future.
Closure
The conclusion of this article serves as an essential ribbon that ties together the exploration of synthetic biology. As we have analyzed various facets, the importance of synthesizing this information cannot be overstated. It not only encapsulates the findings but emphasizes the future directions and implications of the technology.
Summary of Key Insights
Throughout our examination, several key insights emerge. Synthetic biology not only offers innovative solutions in fields like medicine and agriculture but also poses unique challenges. We have seen how genetic engineering techniques such as CRISPR technology and gene synthesis can lead to advancements in treating diseases and enhancing crop resilience. The significant potential for sustainable practices in agriculture highlights the dual focus on productivity and environmental stewardship. Additionally, the ongoing development in cell-free systems and synthetic genomes demonstrates the growing complexity and sophistication within the field. These insights are crucial for scholars and industry professionals, guiding them toward informed decisions in research and application.
The Importance of Ethical Considerations
Addressing ethical considerations is not merely a formality; it is essential for responsible practices in synthetic biology. As advancements in this field progress, ethical frameworks must not lag behind. Issues ranging from biosafety and biosecurity to societal impacts necessitate thorough examination. Stakeholder engagement is critical in addressing public perceptions and assessing risks associated with these biotechnological advancements.