Physical Methods of Microbial Control Explained
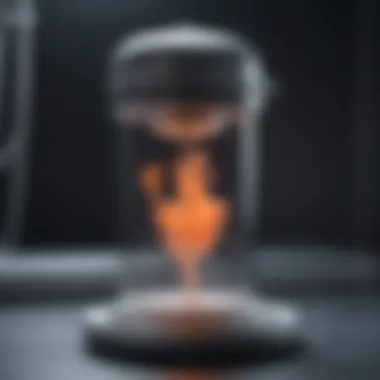
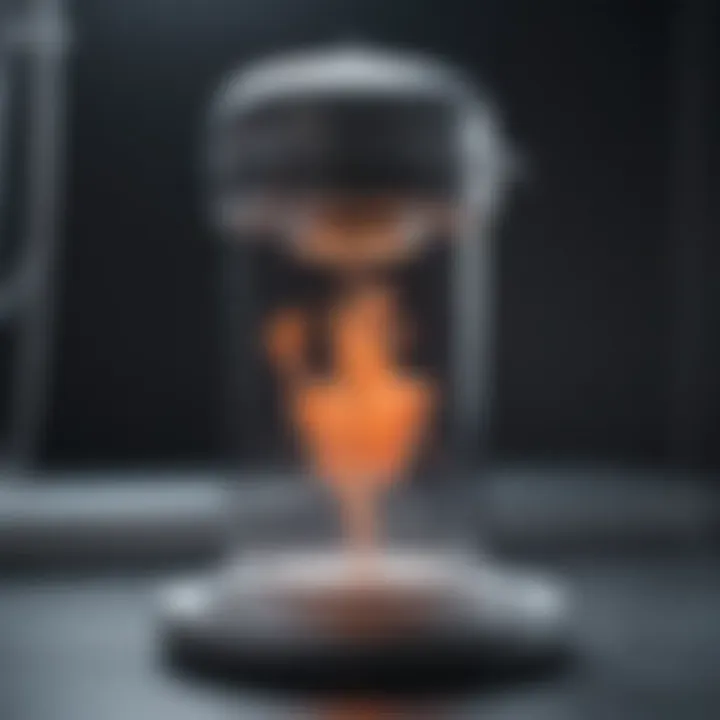
Intro
The control of microbial pathogens is crucial for many sectors, most notably healthcare, food preservation, and environmental safety. Physical methods stand out as viable alternatives to chemical approaches, providing effective solutions for managing microbial populations. Understanding these methods enables researchers and practitioners alike to apply the most suitable techniques in their respective fields. This overview will delve into various physical strategies such as heat, radiation, filtration, and desiccation.
Key Concepts
Definition of Primary Terms
To navigate the discussion, it is important to define some primary terms.
- Microbial Control: Refers to methods used to inhibit or eliminate microorganisms.
- Physical Methods: Strategies that employ physical principles rather than chemical agents to affect microbial growth.
- Desiccation: A process involving the removal of moisture from an environment, often making it inhospitable to microbial life.
These terms set the groundwork for discussing specific techniques, their effectiveness, and their application in various environments.
Related Concepts and Theories
There are several related concepts that provide further insight into the efficiency of physical microbial control methods. For instance, the thermal death time is a critical factor in understanding how heat influences microbial viability. Understanding the mechanisms behind methods such as radiation also draws from principles of microbiology and physics, revealing how microorganisms respond to different wavelengths. Moreover, filtration is rooted in the principles of size exclusion and porosity, which determine the rate and efficacy of microbial removal.
"Physical methods of microbial control leverage natural phenomena, making them integral to sustainable practices in diverse sectors."
Future Directions
Gaps Identified in Current Research
Despite the progress made in exploring physical methods, significant gaps still exist in the research. Many studies focus predominantly on singular methods without thorough comparisons of efficacy across different environments and microbial species.
Suggestions for Further Studies
Future research should aim for a comprehensive evaluation of combined physical methods. For example, studies that investigate the synergistic effects of heat and desiccation could yield valuable insights. Furthermore, long-term studies assessing the durability of these methods in real-world applications would enhance understanding and practical utility.
By articulating these points, this overview not only emphasizes the relevance of physical methods in microbial control but also highlights the need for ongoing research and refinement in these areas.
Prolusion to Microbial Control
Microbial control is a crucial aspect of various fields including healthcare, food safety, and environmental science. This section provides a foundational understanding of microbial control principles and why they are necessary. The rise of antibiotic resistance and the need for effective hygiene practices have underscored the importance of effective strategies to manage microbial populations.
Definition of Microbial Control
Microbial control refers to the methods used to eliminate or manage unwanted microorganisms in a specific environment. This can involve various techniques aimed at reducing the presence of bacteria, viruses, fungi, and parasites. Common goals include the prevention of disease, preservation of food, and maintaining sterile environments in laboratories and hospitals. The terms can cover a range of practices, from reducing microbial load through sterilization to simply monitoring microbial presence.
Importance of Microbial Control in Various Sectors
Microbial control plays a significant role in diverse sectors:
- Healthcare: Controlling pathogens in medical settings is vital to preventing infections, especially in vulnerable populations. Methods such as sterilization and disinfection are routinely used to safeguard patient health.
- Food Industry: In this sector, reduction of spoilage organisms and pathogens ensures food safety and longevity. Techniques such as pasteurization and refrigeration are common practices in food preservation.
- Environmental Science: Microbial control in water and soil helps to maintain ecosystem health. Technologies such as filtration and disinfection ensure clean water supplies.
Understanding these applications aids in appreciating the broader significance of microbial control, as ineffective management can lead to serious health and environmental consequences.
Effective microbial control is not just about eliminating threats; it's about maintaining balance in our ecosystems and ensuring the health of our communities.
Overview of Physical Methods
The focus on physical methods of microbial control underscores a crucial aspect of microbial management. These methods leverage principles that are often more beneficial for specific applications than chemical alternatives. Understanding physical methods provides valuable insights for diverse fields including healthcare, food safety, and environmental science.
In this section, we delve into the distinct concepts of physical methods and their offerings. As chemical methods may introduce various concerns, such as toxicity and environmental damage, physical methods provide a safer and potentially more sustainable option for killing or inhibiting microbes.
What are Physical Methods?
Physical methods refer to techniques used to control microbial growth through non-chemical processes. This includes methods such as heat application, radiation exposure, and filtration. These techniques work by directly influencing the physical state of the microbes, leading to their destruction or growth inhibition.
- Heat: Increased temperature can denature proteins and disrupt cellular functions. Both dry heat and wet heat methods fit into this category, often utilized in sterilization processes.
- Radiation: Ultraviolet and ionizing radiation can damage the DNA and cellular components of microorganisms. These methods are especially significant in sterilizing surfaces and equipment.
- Filtration: By physically removing microbes from air and liquids, filtration methods ensure the sanitation of various materials.
- Desiccation: Removing moisture can lead to microbial dormancy or death, playing a role in food preservation among other applications.
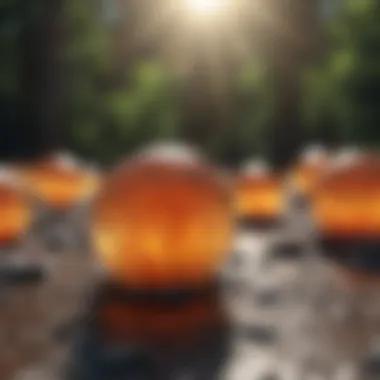
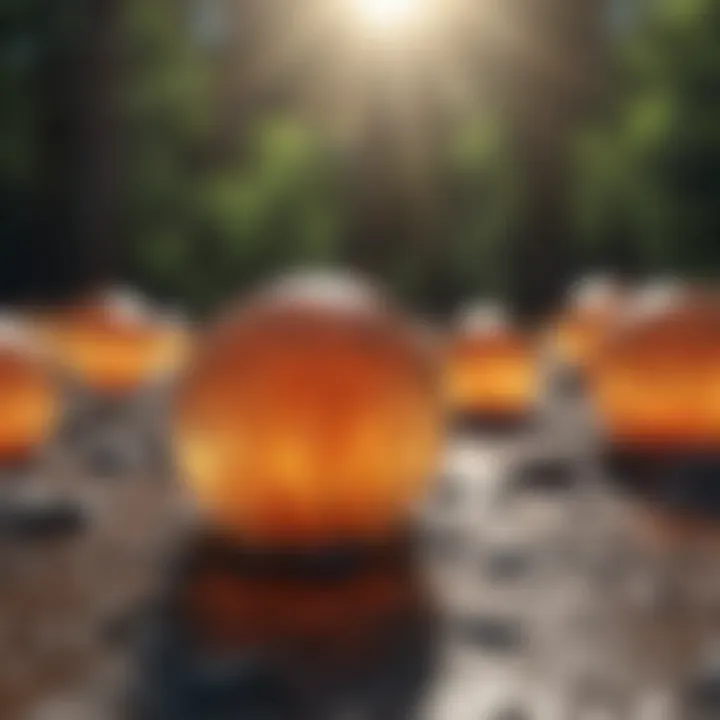
Comparison with Chemical Methods
When comparing physical methods to chemical methods, several factors come into play. Chemical methods often involve the application of substances that can be toxic to both microbes and the environment. This is particularly concerning in fields such as food safety and healthcare. In contrast, physical methods typically present lower risks of harmful side effects.
Key Differences:
- Safety: Physical methods have a lower risk of causing harm to human health and the environment compared to many chemical agents.
- Efficiency: Some physical methods, like heat sterilization, can be more effective under certain conditions, eliminating resistant microbes that chemicals might not affect.
- Residue: Chemical methods may leave residues that can require additional cleaning, whereas physical methods usually do not.
- Adaptability: Physical methods can be tailored for specific applications without the need for intricate chemical formulations.
It is important to consider that while physical methods have notable advantages, they may not always be effective against all types of microorganisms or under all conditions. Ultimately, understanding both physical and chemical methods allows for a more comprehensive strategy in microbial control.
Heat-Based Methods
Heat-based methods provide crucial strategies for microbial control through physical processes. These methods leverage temperature variations to effect changes in microbial viability. Due to their effectiveness, heat methods are widely used across multiple sectors, including healthcare and food preservation. Understanding the nuances of different heat applications is essential for professionals looking to optimize microbial control strategies.
Types of Heat Applications
Dry Heat
Dry heat is characterized by the use of high temperatures without moisture. This method is effective at sterilizing equipment and materials that can withstand high temperatures without degrading. One significant aspect of dry heat is that it can penetrate materials more effectively than wet methods, allowing for complete sterilization of surfaces.
The main advantage of dry heat is its ability to kill microorganisms at high temperatures, typically ranging from 160°C to 180°C. This high temperature ensures that bacterial spores, which are the most resistant forms of life, are effectively eliminated. Additionally, dry heat is often simpler to apply as it does not require specialized equipment for generating steam.
However, there are some disadvantages. For instance, certain materials that cannot tolerate high temperatures, such as some plastics, are not suitable for dry heat sterilization. This limits its applications in specific contexts.
Wet Heat
Wet heat, commonly achieved through steam or boiling water, operates through moisture in conjunction with high temperatures. One critical aspect of wet heat is its effectiveness in killing a broad spectrum of microorganisms, including viruses and fungi. In many instances, wet heat can achieve sterilization at lower temperatures compared to dry heat, making it more energy-efficient.
This method is referred to as moist heat sterilization, and it is often employed in autoclaves, which utilize pressurized steam. The unique feature of wet heat is its ability to penetrate biological materials more quickly due to moisture, resulting in more efficient microbial inactivation.
However, there are certain limitations. Wet heat can damage heat-sensitive materials or degrade specific proteins and enzymes during sterilization, making it unsuitable for all applications. Therefore, careful consideration is essential when selecting the method suitable for a particular material or context.
Mechanisms of Action
The mechanisms through which heat achieves microbial control involve protein denaturation, membrane disruption, and enzyme inactivation. High temperatures affect the structural integrity of proteins, leading to denaturation and loss of function. As proteins are vital for cellular processes, this ultimately causes cell death. Additionally, heat can compromise cellular membranes, increasing permeability and disrupting essential metabolic functions, effectively inhibiting growth or killing the microbe.
Applications in Various Fields
Heat-based methods have diverse applications in various fields:
- Healthcare: Sterilization of surgical instruments, medical devices, and laboratory equipment to prevent infections.
- Food Industry: Pasteurization processes to eliminate harmful microorganisms in food products, such as dairy and beverages.
- Microbiology Laboratories: Autoclaving materials and waste to ensure no viable organisms remain during disposal.
Radiation Methods
Radiation methods play a crucial role in microbial control, leveraging energy waves to eliminate or deactivate microorganisms. Understanding these methods is integral for various fields, such as healthcare, food preservation, and environmental management. These methods utilize specific wavelengths of radiation to disrupt cellular processes in microbes, making them ineffective or nonviable.
Ultraviolet Radiation
Ultraviolet (UV) radiation is a non-ionizing form of radiation commonly used for microbial control. It operates primarily on the principle of damaging the DNA of microorganisms. The specific wavelengths around 254 nanometers are most effective for inactivating bacterial spores and viruses. UV radiation disrupts cellular functions by inducing mutations in the DNA, preventing replication and leading to cell death.
The applications of UV radiation are broad, including water treatment systems, surface disinfection in hospitals, and air purification methods. Despite its efficacy, UV radiation has limitations. Direct exposure can be harmful to human skin and eyes, necessitating protective measures. Additionally, UV effectiveness can be influenced by factors such as microbial load and the presence of particles in water.
Ionizing Radiation
Ionizing radiation, such as gamma rays and X-rays, provides a different approach to microbial control. This form of radiation has sufficient energy to remove tightly bound electrons from atoms, creating ions. This process damages the cellular structures of microorganisms, leading to functional impairments and ultimately cell death.
Ionizing radiation is particularly useful in food irradiation, where it extends shelf life and reduces pathogenic microorganisms. This method is particularly valuable for fruits, vegetables, and meat, offering an alternative to chemical preservatives. However, concerns around food safety and public acceptance of irradiated foods can pose challenges.
Effectiveness of Radiation in Microbial Control
The effectiveness of radiation methods in microbial control lies in their ability to target a wide range of microorganisms, including bacteria, fungi, and viruses. The following points highlight key aspects of their effectiveness:
- Broad Spectrum: Radiation methods can target various pathogens and are effective against resistant strains of bacteria.
- Non-Thermal Action: Unlike heat-based methods, radiation does not change the chemical composition of food significantly, preserving flavor and nutritional value.
- Low Residuals: Radiation does not leave harmful residues, enhancing food safety.
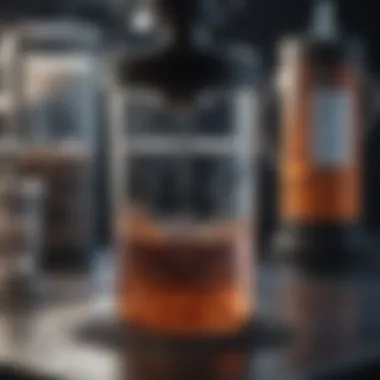
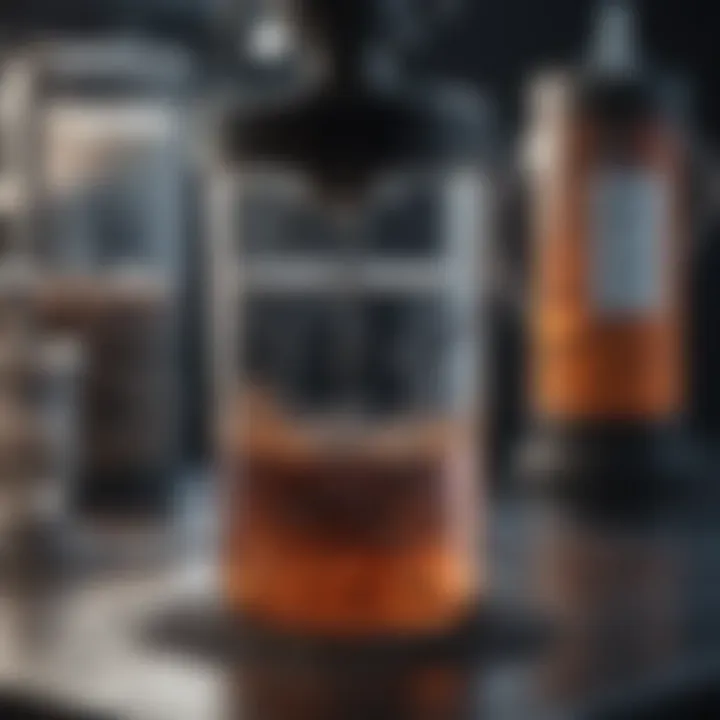
However, effectiveness can be influenced by several factors. The density and type of microbial population, environmental conditions, and the duration of exposure all play important roles. Overall, understanding these mechanisms helps in implementing proper protocols for microbial control in various applications.
"Radiation methods present a viable alternative to traditional chemical approaches by minimizing the risk of resistance and environmental impact."
For further reading, explore more about ionizing and non-ionizing radiation at Wikipedia.
Filtration Techniques
Filtration techniques play a crucial role in microbial control, serving as an effective barrier against unwanted microorganisms. They are particularly important in maintaining sanitation in both water and air purification processes. By employing physical means to separate microbes from liquids or gases, filtration ensures cleaner and safer environments, especially in healthcare and food industries. This section will delve into different types of filtration, their applications, and the limitations associated with them.
Types of Filtration
Membrane Filtration
Membrane filtration is characterized by the use of semi-permeable membranes that allow liquids to pass through while trapping larger particles, including bacteria and viruses. This technique is significant for purifying water and producing sterile solutions in laboratories and industries. Its key advantage lies in its ability to target specific contaminants, making it ideal for critical applications. The unique feature of membrane filtration is its precision; it can achieve a high degree of separation based on size and charge.
However, membrane filtration is not without its challenges. It can be susceptible to fouling, which reduces its effectiveness over time. Regular maintenance is necessary to ensure optimal performance, making it a consideration when assessing cost-effectiveness.
Depth Filtration
Depth filtration involves the use of a thick filter medium that traps particles as liquid flows through it. This method is advantageous for clarifying liquids and has a substantial capacity for holding contaminants. The key characteristic of depth filtration is its ability to accommodate a higher load of particulates compared to surface filters. This makes it a preferred choice for pre-filtration in water treatment processes.
The unique feature of depth filtration is its structure, which allows for multiple layers of filtration. This results in a gradual reduction of particle size, effectively capturing various microbes. Yet, depth filters can have limitations in removing smaller microorganisms and may require additional treatment methods to ensure complete microbial control.
Applications in Water and Air Purification
Filtration techniques are widely applied in water purification systems. They help remove unwanted bacteria, protozoa, and sediments, ensuring that drinking water remains safe and free from contaminants. Similarly, air purification systems utilize filters to trap airborne pathogens, dust, and allergens. Employing effective filtration reduces the risk of airborne diseases and enhances overall air quality, crucial in hospitals and crowded public spaces.
"The efficacy of filtration methods in microbial control cannot be overstated, particularly in sectors where hygiene is a top priority."
Limitations of Filtration Methods
Despite being effective, filtration methods do present limitations. One primary concern is the potential for microbial resistance. Some microorganisms can adapt over time, potentially rendering certain filtration techniques less efficient. Additionally, the effectiveness of filtration is often dependent on the nature and characteristics of the liquid or gas being filtered. In some instances, very small pathogens can pass through certain filtration systems, potentially leading to contamination.
Furthermore, filtration systems can require significant operational costs and maintenance. Understanding these limitations is essential for professionals seeking to implement these methods effectively.
In summary, filtration techniques serve as valuable tools in microbial control, offering various advantages tailored to specific applications. However, ongoing evaluation of their effectiveness and limitations is crucial in ensuring optimal results.
Desiccation and Its Role in Microbial Control
Desiccation, or the removal of moisture, is a critical method in microbial control. This method leverages the natural vulnerability of microorganisms to dehydration. Its significance lies not only in its efficiency in managing microbial growth but also in its application across several industries, particularly food preservation. Understanding desiccation provides insights into its strategic advantages and challenges.
Mechanisms of Desiccation
The process of desiccation mainly involves creating an environment devoid of water, which is essential for microbial survival. Microorganisms, including bacteria, fungi, and viruses, rely on moisture for metabolic processes. When they encounter a drastically reduced water availability, several mechanisms of action are triggered:
- Osmotic Pressure: The loss of water leads to a loss of turgor pressure in microbial cells. This pressure drop can cause cell lysis or inhibit cell division.
- Metabolic Disruption: Without water, biochemical reactions slow down or stop entirely, leading to eventual cell death.
- Protein Denaturation: Proteins, which require hydration for their correct functioning, become denatured. This can result in a breakdown of essential cellular functions.
Desiccation is effective because it exploits these fundamental aspects of microbial life. However, some microorganisms, like spores, can survive extreme dryness, which necessitates careful consideration in application.
Applications in Food Preservation
Desiccation finds extensive use in the food industry as a preservation technique. This approach is particularly important in extending shelf life and maintaining food quality. Key applications include:
- Dried Fruits and Vegetables: Removing water from these products prevents microbial growth that can lead to spoilage.
- Jerky and Other Dried Meats: The dehydration process not only preserves these meats but also adds unique flavors.
- Powdered Milk and Eggs: These are made shelf-stable through desiccation, providing long-lasting options for consumers.
- Freeze-Dried Meals: Often used in camping and military contexts, the freeze-drying process preserves meals by removing moisture without heating, maintaining nutrients and flavors.
The food industry benefits from desiccation not only for preservation but also for logistical reasons. Desiccated products are often lighter and require less packaging.
"Desiccation not only significantly enhances the longevity of food products but also retains their sensory properties when done correctly."
Advantages of Physical Methods
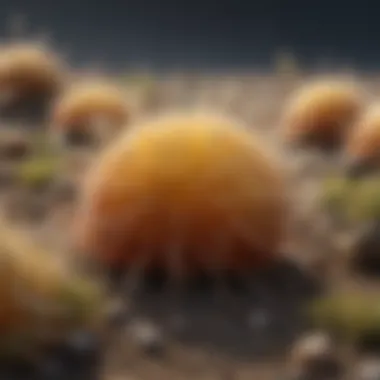
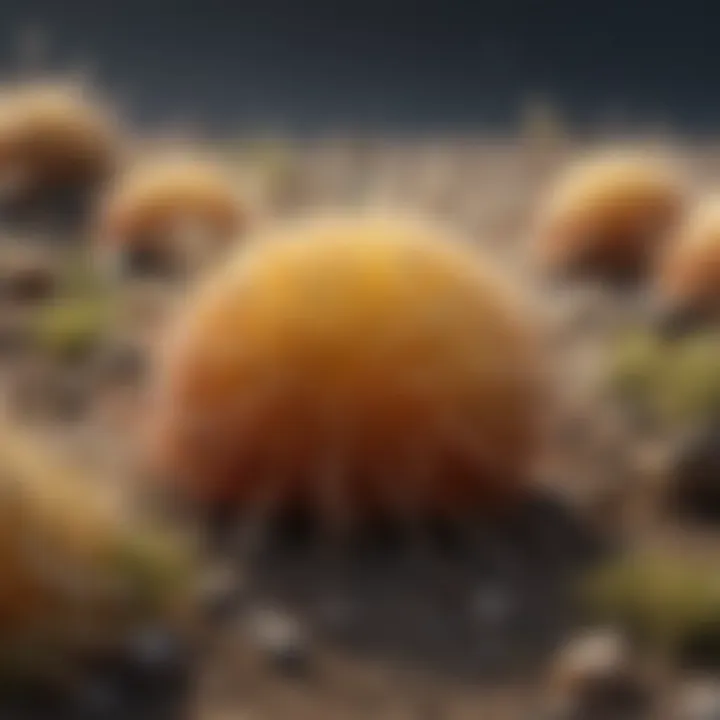
Physical methods of microbial control offer several compelling advantages over their chemical counterparts. Their significance in various applications, such as healthcare, food safety, and environmental management, cannot be understated. Understanding these advantages helps in making informed decisions regarding the best practices for microbial control, as well as promoting safer and more sustainable practices overall.
Safety and Environmental Considerations
One of the most important advantages of physical methods is their inherent safety profile. Unlike chemical agents, which may introduce toxic residues into the environment, methods such as heat, radiation, and filtration generally do not leave harmful by-products. This is especially crucial in settings where the health of vulnerable populations is at stake, such as hospitals and food production facilities.
Moreover, the emphasis on safety extends to the workers who implement these methods. For instance, using ultraviolet radiation for disinfection can be managed with appropriate shielding, reducing exposure risks for personnel. The absence of harmful chemicals not only safeguards human health but also protects ecosystems from the potential negative impacts of chemical runoff or spills.
In addition to safety, physical methods are also often more environmentally friendly. Techniques such as desiccation or heat treatments do not contribute to air pollution or soil contamination. They provide a cleaner alternative that aligns with the increasing emphasis on sustainability in microbial control strategies.
"Physical methods reflect a shift towards sustainability and safety in microbial control, reinforcing a proactive approach to public health and environmental protection."
Cost-Effectiveness
Cost-effectiveness is another defining factor in the advantages of physical methods. While the initial investment in equipment like autoclaves or UV sterilizers may be significant, these tools typically offer long-term savings. Physical methods often require lower operational costs over time, providing a favorable return on investment compared to the recurring expenses associated with chemical disinfectants.
Additionally, the effectiveness of physical methods can reduce waste disposal costs. Unlike chemical agents, which may require special handling and disposal due to environmental regulations, physical methods do not usually create hazardous waste. This means organizations can often navigate compliance with fewer expenses and more straightforward processes.
Physical methods also tend to require less frequent application than chemical treatments, which can lead to a more streamlined approach. For example, effective heat treatment can render surfaces and instruments sterile in a single operation, whereas chemical disinfectants may need repeated applications. Overall, the initial costs and operational efficiency point to a cost-effective approach that can benefit various sectors.
In summary, the advantages of physical methods are evident in their safety, environmental friendliness, and cost-effectiveness. Exploring these benefits provides essential insights for researchers and professionals in their pursuit of effective microbial control strategies.
Limitations and Challenges
Understanding the limitations and challenges associated with physical methods of microbial control is crucial for effectively deploying these techniques. While these methods offer several advantages such as safety and environmental friendliness, they are not without their drawbacks. Identifying these limitations allows researchers and practitioners to make informed decisions when selecting appropriate microbial control measures in various contexts. This section highlights some significant specific elements concerning resistance to methods and application restrictions that require careful consideration.
Resistance to Methods
Resistance to physical methods of microbial control presents an ongoing challenge. Microorganisms can sometimes adapt to environmental stressors, leading to reduced efficacy of techniques like heat and radiation. The phenomenon of resistance can manifest in several ways:
- Thermal resistance: Some bacteria can form heat-resistant spores. These spores become inactive at high temperatures but can reactivate when conditions improve, complicating control efforts.
- Radiation tolerance: Certain organisms have mechanisms that allow them to repair DNA damage caused by UV or ionizing radiation. This ability can lead to survival despite exposure to levels that should be lethal.
Efforts to address resistance are continually evolving. Researchers focus on understanding the underlying mechanisms of microbial resistance and developing strategies to overcome it. This might include combining different physical methods to target the same population effectively, thereby reducing the chance of survival and adaptation.
Application Restrictions
Application restrictions further limit the effectiveness of physical methods in microbial control. These restrictions can be attributed to several factors, including:
- Material compatibility: Some methods, particularly heat and radiation, may not be suitable for all materials. For example, heat can degrade plastics or other sensitive components, while UV radiation can only penetrate surfaces to a limited degree.
- Scale of application: While certain methods can effectively eliminate microbes on a small scale, scaling these approaches to larger environments or volumes may present difficulties. For example, ensuring uniform heating in bulk food processing can be challenging.
- Environmental conditions: Specific physical methods are dependent on environmental factors. High humidity levels can hinder effective desiccation, while varying light conditions can influence the effectiveness of UV radiation in microbial control.
Recognizing these restrictions can guide practitioners in selecting appropriate methods depending on the setting and type of microbial contamination present.
It is essential to consider these limitations and challenges when implementing physical methods of microbial control, to increase the overall effectiveness and safety of such strategies.
Epilogues and Future Directions
The conclusions drawn from examining physical methods of microbial control reflect a crucial aspect of current and future strategies in microbial management. Understanding these methods not only assists in mitigating microbial risks but also shapes development in various sectors such as healthcare, food preservation, and environmental protection. The continuous evolution in microbial control presents both challenges and opportunities.
Emerging trends indicate a shift towards more sophisticated approaches that leverage advancements in technology. As we deep dive into these advancements, it becomes essential to acknowledge the growing complexity of microbial ecosystems. This means that the ways we approach microbial control need to be adaptive and multifaceted to ensure effectiveness.
Emerging Trends in Microbial Control
Recent research highlights several trends altering the landscape of microbial control:
- Nanotechnology: Use of nanoparticles has shown promise in enhancing the efficacy of antimicrobial agents.
- Bioengineering: Genetic manipulation allows for the creation of microbes that can actively suppress pathogens.
- Smart Materials: Development of surfaces that can autonomously kill microbes upon contact is gaining traction.
- AI and Data Analytics: Increased integration of artificial intelligence to predict microbial behavior can lead to targeted interventions.
These trends suggest a more integrated approach to microbial control that not only utilizes physical methods but also incorporates technological advancements to maximize effectiveness.
Integration of Physical and Chemical Methods
The integration of physical and chemical methods presents a synergistic opportunity for improved microbial control. By combining both types, the limitations of one can be offset by the strengths of another. For instance:
- Complementary Mechanisms: While heat can denature proteins, a chemical agent might work to disrupt DNA, providing a robust approach to eradication.
- Enhanced Effectiveness: Using a combination can lower the necessary concentrations of chemical agents, thereby reducing potential side effects and resistance development.
- Broader Application Range: Certain environments may be better suited for one method as opposed to the other; integration allows for flexibility in varying conditions.
"A multifaceted approach is the key to effective microbial control in diverse contexts, combining various methods where each excels."
Looking ahead, future directions in microbial control will likely center on refining these integrations and leveraging emerging technologies. There is a clear need for ongoing research that not only evaluates new methodologies but also critically assesses their impact on public health and safety.
Overall, the challenges facing microbial management are significant, but so are the innovations on the horizon. The commitment to exploring and implementing diverse and complementary strategies will be pivotal in shaping effective microbial control practices.