Current Trends in Physical Science Research

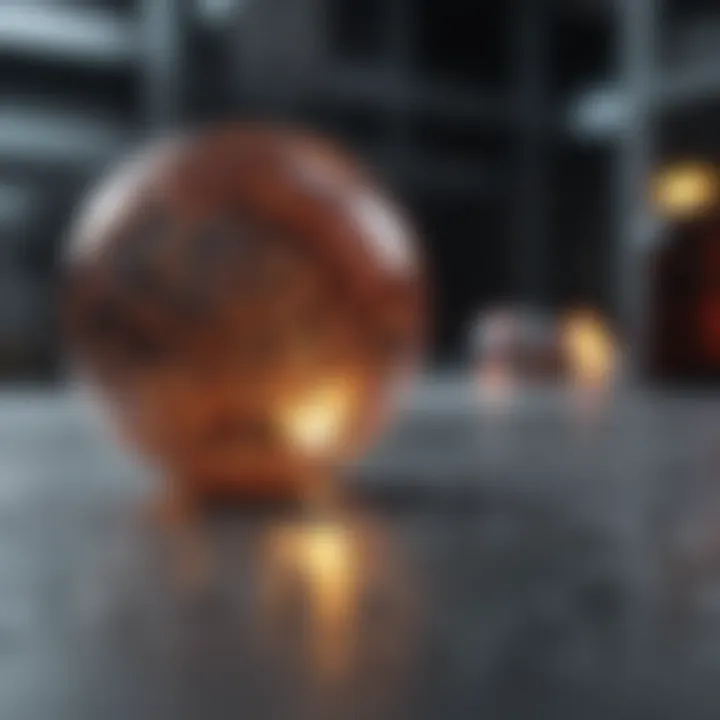
Intro
In the vast expanse of the physical sciences, researchers explore an impressive array of topics, each contributing to our understanding of the universe. The synergy between established disciplines and emerging areas of study fosters continuous innovation, adapting to societal needs and technological advancements. From materials science, where the fabric of our everyday lives is weaved, to the subtleties of quantum mechanics that hint at a more intricate reality, these fields are not merely academic pursuits; they shape the future.
As we embark on this exploration of the current landscape in physical science research, it’s essential to grasp fundamental concepts. This article aims to unveil core themes like advancements in materials science and energy sustainability, while also shedding light on the pivotal role of quantum mechanics. Digging deeper, we’ll highlight various sub-disciplines and methodologies that are defining research today, addressing not only what is being studied but also how these efforts intertwine with the broader narratives of science and technology.
The importance of staying abreast with the latest advancements cannot be understated, particularly for academics, students, and industry professionals alike. Understanding these dynamics helps in shaping future research endeavors and innovative solutions to pressing global challenges.
"The beauty of physical science lies in its quest not only for knowledge but for practical applications that can lead to significant societal advancements."
As we unfold this discussion, the sections to follow will provide a thorough examination of key concepts and future directions, offering insights into both the triumphs and the hurdles faced by researchers today.
Prelims to Physical Science Research
Physical science research serves as the bedrock of our understanding of the natural world, laying the foundation for advancements that ripple through countless domains. It encompasses a broad spectrum of disciplines, from the fundamental laws of physics to the intricate interactions of chemical compounds. This article aims to explore these diverse topics and illuminate the contemporary trends that define physical science today.
Research in this field is not merely academic; it drives innovation and contributes to societal needs—be it through developing sustainable energy sources or deciphering the complexities of matter. By investigating such themes, students, researchers, and educators can gain perspective on how various elements interconnect, shaping both scientific inquiry and applied practices.
Going beyond rote learning, it is essential to grasp how physical sciences inform real-world challenges. For instance, evaluating the climatic shifts requires insights from earth sciences, while medical breakthroughs often hinge on principles from physics and chemistry. In the ensuing sections, a detailed examination of key topics will highlight the multidimensional nature of physical science research and demonstrate its profound significance.
Definition and Scope
Physical science research can be defined as a systematic study focused on understanding the nature and properties of the physical universe. This research typically involves observation, experimentation, and theoretical modeling. The scope is vast, encompassing disciplines such as physics, chemistry, earth sciences, and astronomy. Each of these disciplines contributes unique methodologies and perspectives, allowing scientists to address various questions—from atomic behavior to cosmic phenomena.
Each discipline plays a vital role in exploring phenomena such as:
- Physics: Examining forces and energy.
- Chemistry: Understanding substances and their reactions.
- Earth Sciences: Analyzing natural processes and their impacts.
- Astronomy: Investigating celestial mechanics and cosmology.
These areas are interconnected; knowledge gained from one can significantly enhance understanding in another. This integration of disciplines fosters collaboration, making the research process rich and fruitful.
Significance in Modern Science
The significance of physical science research in modern times is manifold. It underpins technological advancements that improve our quality of life, from healthcare innovations to cleaner energy solutions. In today’s context, challenges such as climate change and public health crises can only be effectively addressed through the insights derived from physical sciences.
Moreover, the role of physical science is intertwined with:
- Global Collaboration: Research transcends borders, leading to international partnerships that pool resources and expertise.
- Technological Development: Continuous advancements often hinge on discoveries within physical science, from new materials to enhanced information technology.
- Policy Making: Scientific evidence shapes decisions about environmental protection, resource management, and public health initiatives.
"Physical science is the gateway to understanding our universe; it is not only about discovering phenomena, but also about forging the path towards a sustainable future."
As the world faces unprecedented challenges, the significance of physical science becomes increasingly clear. Both foundational knowledge and innovative applications are critical, illustrating why research in this domain must remain a priority for academic institutions, governments, and industries alike.
Core Disciplines of Physical Science
In the landscape of physical science, core disciplines serve as the bedrock upon which intricate theories and applications are built. These fields are crucial not only for their historical significance but also for their ongoing contributions to understanding the universe and contributing to technological advancements. By exploring core disciplines, we can delve into the fundamental laws governing our world, the intricacies of materials, and even the vastness of space itself. Each core discipline plays a distinct yet interconnected role, providing valuable insights into how matter and energy interact.
Physics: Laws and Theories
Physics stands at the forefront of natural sciences, encompassing the study of matter, energy, forces, and the fundamental laws that govern them. Physicists seek to understand the basic principles of the universe, from the motion of particles to the phenomena of black holes. When we talk about laws like Newton's laws of motion or Einstein's theory of relativity, we're discussing frameworks that help us interpret vast arrays of observations. Physics not only addresses classical mechanics but also delves into statistical mechanics, thermodynamics, and quantum mechanics, each opening doors to groundbreaking technologies.
For instance, advancements in quantum mechanics have led to developments in quantum computing and cryptography, radically changing how we approach processing and sharing information. This discipline’s significance cannot be overstated; it forms the backbone of modern technology and our grasp of the universe.
Chemistry: Composition and Reactions
Chemistry, the science of matter and its transformations, directly connects to almost every aspect of our daily lives. It examines substances, their properties, and how they interact, combine, and change. Understanding chemical compounds is essential, whether developing new materials, creating pharmaceuticals, or tackling environmental issues like pollution.
Consider the implications of synthetic chemistry in creating sustainable materials. Innovations such as biodegradable plastics or catalysts that minimize waste during chemical processes reflect the discipline's relevance to our modern challenges. The crossover between chemistry and technology helps to address pressing global concerns, fostering advancements that enhance both human life and the environment.
Earth Sciences: Processes and Phenomena
Earth sciences encompass the study of the Earth and its various systems, including geology, meteorology, oceanography, and ecology. This field is paramount in understanding the processes that shape our planet, from tectonic shifts and weather patterns to ocean currents and ecosystems. Having knowledge of these processes is essential for addressing natural phenomena, such as earthquakes or climate change impacts.
An illustrative example of earth science's importance is the modeling of climate change. Researchers utilize geological data and climate models to predict future conditions and inform policy decisions. This discipline equips us to respond to environmental challenges, shaping sustainable practices for a better future.
Astronomy: Celestial Bodies and Their Movements
Astronomy takes us beyond the Earth, exploring the vast universe and our place within it. This discipline investigates celestial bodies, their movements, and the fundamental forces that govern them. From the observation of stars and planets to the study of cosmic phenomena such as supernovae and black holes, astronomy broadens our understanding of the universe.
The relevance of astronomy extends to practical applications like satellite technology for navigation and communication. Space exploration missions, such as those undertaken by NASA or the European Space Agency, have yielded advancements in technology and materials that find uses even in everyday life.
"The cosmos is within us. We are made of star-stuff. We are a way for the universe to know itself."
— Carl Sagan
Overall, the core disciplines of physical science not only unravel the mysteries of existence but also hold the key to our future innovations and solutions in various fields of society.
Recent Innovations in Physical Sciences
Recent developments in physical sciences have propelled research and technologies into unexplored territories. This section zeros in on how these innovations not only push the boundaries of known science but also create new vistas for practical applications. They hold the key to addressing some of today's most pressing global challenges. These innovations pave the way for solutions that can improve lives, alter industries, and spark academic interest. Each subset of innovations represents a mosaic of collaborative efforts, showcasing how crucial interdisciplinary dialogue is in fostering advancement.
Nanotechnology Advances
Nanotechnology has emerged as a game changer in various sectors, creating materials and devices on a nanometer scale. This tiny scale expands possibilities. For instance, nanoparticle-enhanced sunscreens can improve UV protection without leaving a grease mark on the skin, an application that’s not only practical but innovative.
Another fascinating development is the use of nanomaterials for drug delivery. By tethering drugs to nanoparticles, researchers can target specific cells in the body, ensuring treatments work more efficiently. The success in medical applications highlights how manipulation at nanoscale can lead to precisely tailored therapies.
"Nanotechnology signifies a quantum leap in our approach to not just enhancing existing products but also creating new opportunities for societal benefits."
With applications in electronics, energy storage, and medicine, the continuing breakthroughs in this field are of paramount importance.
Progress in Materials Science
Materials science is another domain that has witnessed serious advancements. Researchers are uncovering new compounds and composites that change the game completely. For example, materials with shape memory, like certain alloys that can revert to their original form after deformation, are increasingly useful in robotics and biomedical devices, demonstrating their versatility.
Additionally, developments in 2D materials, like graphene, are sparking immense interest. This ultra-thin material boasts extraordinary conductivity and strength. The research surrounding it holds vast promise for applications in flexible electronics and energy storage solutions, making it a hot topic in scholarly circles.
Considerations around sustainability have also taken center stage in materials science, leading to increased efforts in developing eco-friendly materials. Innovations such as biodegradable plastics can help alleviate environmental concerns and represent an essential area of focus, often linked to economic and social implications.
Breakthroughs in Renewable Energy Research
As the world wrestles with climate change and depleting fossil fuels, renewable energy research stands at the forefront. Solar energy is witnessing significant advancements, especially with perovskite solar cells, which promise to deliver higher efficiency at a lower cost. The adaptability of these materials in various environments offers diverse applications, potentially accelerating the shift to sustainable energy.
Wind energy also benefits from advancements in turbine design and aerodynamics, resulting in more efficient energy harvesting. Ongoing research seeks to optimize the placement of wind farms to maximize output, as studies suggest that careful geographic planning can yield significant increases in energy production.
Lastly, energy storage technologies are making strides. Innovations in battery technology, such as solid-state batteries, have the potential to increase energy density and decrease charging times, addressing a significant bottleneck in deploying renewable energy on a larger scale.
In summary, recent innovations in physical sciences speak to the ingenuity and resolve of the research community. The focus on nanotechnology, materials science, and renewable energy underlines not just the advancements in each field but also the potential for cross-disciplinary applications. Moving forward, these ongoing explorations are vital in tackling our modern challenges, laying the groundwork for a more sustainable and technologically advanced future.
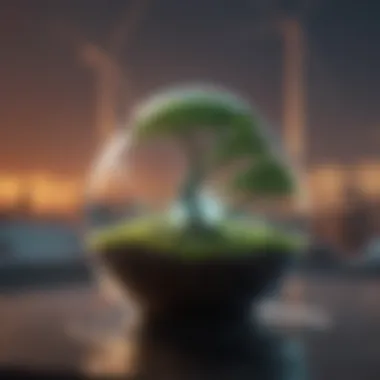
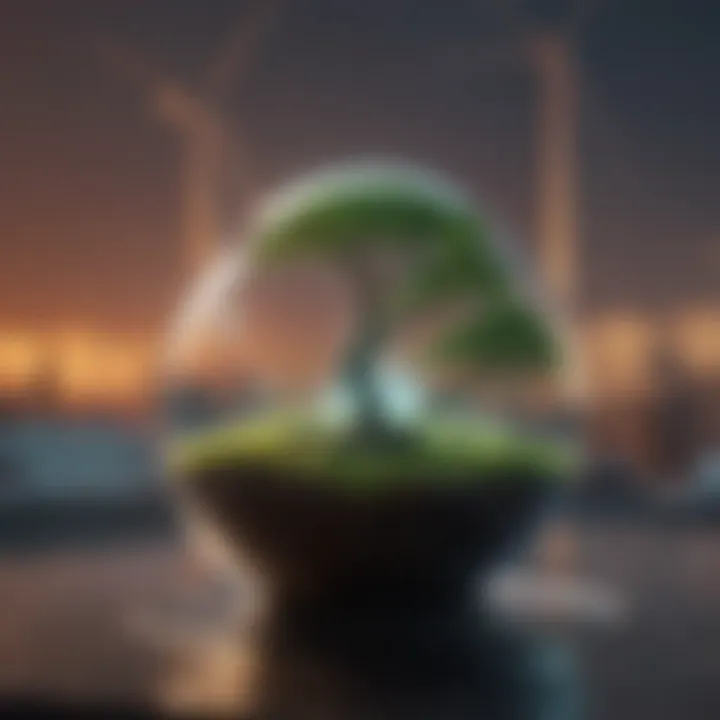
Emerging Research Areas
Emerging research areas in the field of physical sciences represent the landscape of innovation and scientific curiosity that is continually evolving. Such topics not only illuminate new frontiers but also provide the necessary framework for addressing modern-day challenges. As issues like climate change, health crises, and technological advancement grow increasingly complex, the role of interdisciplinary collaboration becomes paramount. Exploring these areas allows researchers and practitioners to leverage combined expertise, leading to more robust solutions and fostering a creative environment rooted in shared knowledge.
Interdisciplinary Research
Interdisciplinary research is the cornerstone of many emerging developments in physical science. It involves integrating methods and concepts from various disciplines to tackle complex problems. For instance, the collaboration between physicists, chemists, and biologists on nanotechnology projects illustrates how diverse perspectives can intersect, enhancing innovation. In this approach, there is an increased ability to harness the strengths of different fields, allowing for a more holistic view of phenomena and drawing upon methodologies that would otherwise remain isolated.
Furthermore, interdisciplinary teams often yield unique insights, addressing research questions more thoroughly. An example is the work of environmental scientists who merge climatology, ecology, and energy studies to formulate sustainable solutions. This assimilation of knowledge not only fosters meaningful progress but also creates rich academic environments that encourage students and young professionals to broaden their scopes.
Quantum Computing and Its Implications
Quantum computing stands out as a leading frontier in technological advancement. This area has gained traction due to its potential to solve complex problems that traditional computers cannot handle efficiently. It employs principles of quantum mechanics to process information in ways that challenge classical understanding.
The implications of quantum computing are vast, affecting various sectors ranging from cryptography to pharmaceuticals. For example, quantum algorithms can analyze enormous datasets at unprecedented speeds, paving the way for breakthroughs in drug discovery by identifying molecular interactions that classical computing struggles with.
"Quantum computing is not just another technology; it is a paradigm shift that can redefine problem-solving frameworks across fields."
Moreover, the ethical considerations surrounding this technology are critical. Questions about data privacy, security, and the socio-economic impacts of such advancements warrant examination from a plethora of perspectives. Researchers must engage in thoughtful discourse on how to navigate these challenges while promoting responsible development.
Environmental Monitoring and Data Analysis
In today’s world, the significance of environmental monitoring cannot be overstated. The interplay between technological innovations in data analysis and the need for sustainable practices forms a vital area of focus in physical science research. Environmental scientists utilize various methods ranging from satellite imaging to sensor networks for comprehensive data collection on climate patterns, pollution levels, and biodiversity.
Through sophisticated data analysis techniques, such as machine learning, researchers can identify trends, predict future events, and inform public policy. This synergy between data science and environmental efforts enables proactive responses to pressing issues like natural disasters, resource depletion, and ecosystem health.
The shift toward cloud computing has further enhanced data analytics. Researchers can now access vast datasets globally, fostering collaboration. By employing tools that analyze data patterns, scholars can produce insightful forecasts that aid in conservation strategies and urban planning.
In summarizing the growing importance of emerging areas within physical science, it is clear that they hold the key to addressing significant global challenges. Such interdisciplinary cooperation, advancements in quantum computing, and rigorous environmental analytics not only enrich the scientific community but also pave the way for meaningful societal impact.
Methodologies in Physical Science Research
In the ever-evolving field of physical science, methodologies are the backbone of any research initiative. They dictate how projects are conducted, ensuring that findings are valid, reproducible, and meaningful. From experimental design to computational techniques, each methodology serves distinct purposes at different phases of research. A clear understanding of these methodologies is crucial for students, researchers, and professionals, as improper application or misunderstanding could lead to flawed conclusions or wasted resources.
Experimental Design
At the foundation of scientific inquiry lies experimental design. The process involves structuring an experiment to test hypotheses effectively while controlling external variables. A well-thought-out design allows researchers to make sense of data and draw substantial conclusions.
- Defining Objectives: The first step in designing an experiment is isolating what you hope to learn. This sets the stage for a focused investigation.
- Control Groups: Including control groups is pivotal. It serves as a baseline against which experimental results can be compared. Knowing whether the changes observed are due to the manipulation of a variable or just random fluctuations in data can make all the difference in the integrity of the study.
- Randomization: Incorporating randomization helps to eliminate bias. This way, each participant or data set has an equal chance of being assigned to any group, leading to more reliable results.
Through thoughtful experimental design, studies in physical science can yield insightful data that could lead to significant advancements.
Computational Techniques
In the digital age, computational techniques have become indispensable in elucidating complex problems in physical science. They allow for extensive data analysis, modeling, and predictions that would be nearly impossible to achieve otherwise. Sophisticated algorithms enable researchers to simulate systems, analyze vast quantities of data, and visualize outcomes.
- Modeling Systems: For example, researchers often use simulation software to investigate molecular dynamics or predict particle behavior under varying conditions. The ability to model scenarios helps in drawing parallels with real-world phenomena.
- Machine Learning Applications: Advanced techniques like machine learning are increasingly being utilized to detect intricate patterns within data sets that experts might overlook.
- Data Analysis: Statistical analysis software assists in managing large data sets, enabling scientists to explore relationships and trends that inform their hypotheses.
Adopting computational techniques not only accelerates progress but also enhances the precision of conclusions drawn in physical science.
Field Studies and Observational Research
Field studies and observational research provide another layer of insight, especially in understanding real-world phenomena. Unlike controlled experiments performed in labs, these methods focus on observing and collecting data in the natural environment, offering a rich context for scientific inquiry.
- Practical Applications: For instance, scientists studying climate change collect data from various environments, helping to understand broader climate trends that can’t always be replicated in a lab.
- Longitudinal Studies: These studies involve repeated observations over time, providing insights into long-term trends and changes in natural systems. They can reveal patterns that single-point studies might miss.
- Local Knowledge: Engaging with local populations can also enrich field studies, as indigenous knowledge often provides context or insights into ecological changes or sustainable practices.
Engaging in field studies allows scholars to grasp the nuances of complex systems, ensuring that findings are grounded in the realities of the world.
"Methodologies are not just tools; they are the lenses through which we view and interpret the natural world. Understanding their application is crucial in advancing physical science."
Critical Challenges in Physical Science Research
In the landscape of physical science research, several critical challenges demand attention. These issues not only impede the progress of scientific inquiry but also influence how research is conducted and funded. Understanding these challenges is crucial for students, educators, researchers, and professionals who are keen on navigating the complex web of physical sciences.
Funding and Resource Allocation
One of the foremost challenges physicists and other scientists face is securing adequate funding. Research in physical sciences can require substantial financial resources for equipment, personnel, and materials. In many cases, the competition for grants is fierce, often leaving deserving projects unfunded. Institutions like the National Science Foundation (NSF) and similar organizations play a pivotal role in providing such funding. However, they often prioritize projects that promise high visibility or immediate returns.
In light of these financial constraints, many researchers adopt creative strategies to maximize their resources. Collaborative projects that pool resources from multiple institutions can be an effective way to increase funding potential. Additionally, establishing partnerships with industry can provide alternative funding streams, although these may come with certain expectations regarding research outcomes.
A few key considerations include:
- Diversity of Funding Sources: Seeking funding from a mix of public grants, private investment, and crowdfunding.
- Budget Justification: Clearly articulating why financial support is necessary for specific facets of the research.
- Long-term Planning: Developing a funding strategy that aligns with the pace and goals of the research.
Reproducibility Crisis
The reproducibility crisis in the scientific community is another pressing concern. Numerous studies have revealed that many experiments, especially in the physical sciences, cannot be replicated successfully. This situation raises serious questions about the validity of research findings and has sparked debates about the integrity of scientific practices.
Some contributing factors to the reproducibility crisis include:
- Lack of Standards: The absence of standardized methodologies can result in varied interpretations and applications of experiments.
- Publication Bias: Journals tend to favor positive or novel results, which may lead to an underrepresentation of negative findings that could inform best practices.
- Pressure to Publish: In academia, there’s often a push to continuously publish results, sometimes at the expense of rigorous experimental protocols.
Addressing these issues is crucial not just for the credibility of the research but also for the scientific progress as a whole. Encouraging transparency and open data sharing can aid in mitigating the crisis.
Ethics in Research Practices
Another key area of challenge revolves around ethics in research practices. Researchers must navigate a delicate balance between pushing boundaries to discover new knowledge and adhering to ethical standards. Issues such as data manipulation, plagiarism, and the treatment of human subjects are paramount in this discussion.
The ethical landscape can be complex, particularly as technology evolves. Online data repositories and the ease of access to information raise questions about data ownership and integrity. Key ethical considerations include:
- Informed Consent: Ensuring participants are fully aware and agree to their involvement in research.
- Data Integrity: Upholding standards to prevent falsification of results, which can have widespread implications.
- Environmental Responsibility: Researchers must consider the environmental implications of their work, especially in fields that intersect with ecological sustainability.
Researchers are encouraged to engage with various ethical boards and committees to ensure their work aligns with established ethical guidelines and respects societal norms.
"The foundation of progress in science rests not only on discoveries, but on the ethical dimensions of those discoveries."
The Role of Technology in Research Enhancement
The advancement of technology has become a linchpin in the evolving landscape of physical science research. Its influence permeates various disciplines, enhancing methodologies and transforming the way researchers approach complex problems. By leveraging cutting-edge tools and systems, scientists can achieve unprecedented levels of precision, efficiency, and creativity. This section will explore key technology facets that bolster research efforts, such as data analysis tools, simulation software, and laboratory automation.
"Since the inception of modern science, technology has played a pivotal role in research, but today, it's the very backbone of innovation."
Data Analysis Tools
Data analysis tools provide the means to sift through vast amounts of data, allowing researchers to derive meaningful insights from their findings. In this digital age, the sheer volume of data generated is staggering. Traditional methods of analysis often fall short, leading to potential misinterpretations. Researchers today rely on both specialized software, like MATLAB or Python libraries, and machine learning algorithms to process and analyze their datasets.
- Statistical Tools: Programs such as R or SPSS allow for sophisticated statistical analysis, which is vital in validating hypotheses or examining experimental results.
- Visualization Software: Tools like Tableau or D3.js can present data in an accessible format, which aids in communicating complex findings to both scientific peers and the general public.
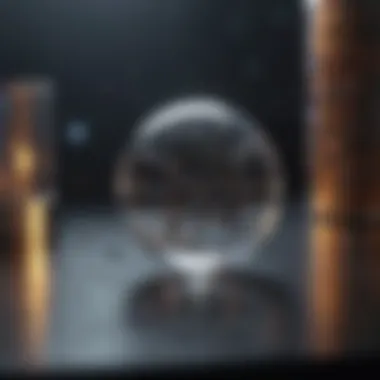
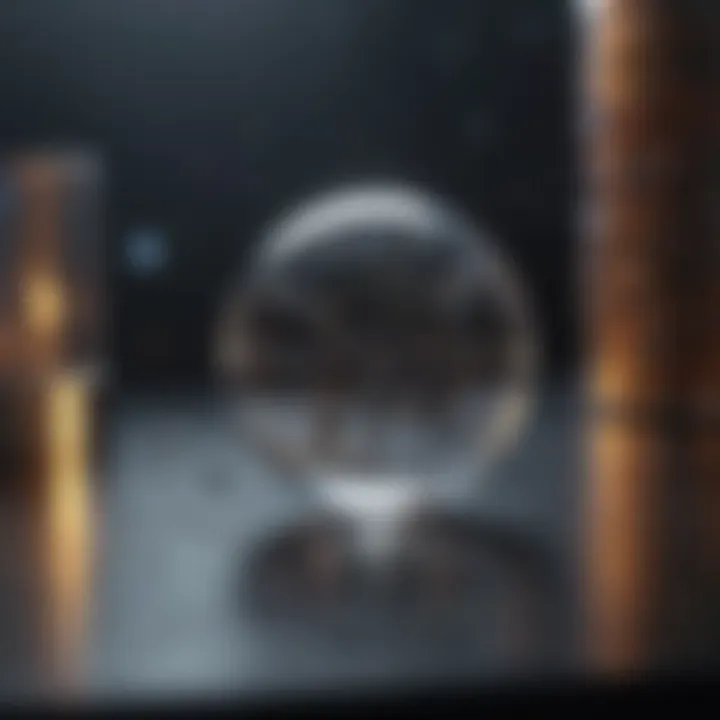
Utilizing these tools can assist in identifying patterns and correlations that might otherwise go unnoticed. It also fosters collaborative efforts across disciplines, as shared data can be interpreted through diverse lenses.
Simulation Software
Simulation software serves as a virtual playground for researchers, enabling them to model physical phenomena and test theories in a controlled environment. This not only saves time and resources but also allows for error minimization before any real-world applications are attempted.
- Physics Simulators: Programs like COMSOL Multiphysics provide researchers with the ability to simulate different physical conditions without the costs of extensive laboratory setups.
- Interdisciplinary Applications: In fields such as materials science, simulators can predict how materials will behave under various conditions, leading to faster innovation cycles.
By using simulation software, researchers gain insights that help guide experimental design. They can also explore scenarios that may be impractical or impossible to recreate in reality.
Laboratory Automation
Gone are the days when research labs seemed like a messy affair filled with manual tasks. Laboratory automation has revolutionized data collection and experimental procedures, significantly speeding up experiments while minimizing human error.
- Automated Liquid Handling: Devices that can transfer precise volumes of liquids free researchers from the tedious task of pipetting, which allows for increased throughput.
- Robotics and AI: The integration of robotics in laboratory settings has led to developments where robotic arms can perform complex experiments autonomously. Combining this with AI can lead to self-optimizing experiments that learn and adapt on the fly.
The paradigm shift towards automation in labs not only boosts efficiency but also frees human intellect for more creative and analytical tasks.
Thus, the role of technology in enhancing research is both expansive and critical. With each new tool, researchers are equipped to tackle challenges with renewed vigor, ultimately forwarding the collective knowledge within the physical sciences.
Impact of Global Issues on Physical Science Research
The interplay between global issues and physical science research is becoming increasingly vital in understanding our world. The challenges we face—be it climate change, health crises, or the advancement of technology—shape the landscape of research priorities, funding, and collaborative efforts across disciplines.
Here, we will explore the profound implications of these global issues on physical science research, focusing on how researchers adapt their strategies, and the benefits that arise from such adaptations.
Climate Change and Its Effects
Climate change stands as a monumental challenge of our times. Scientists are tasked with seeking not just understanding but also solutions to mitigate its destructive impacts. Physical science plays a critical role in areas such as atmospheric studies, materials development, and energy transitions.
- Emerging Data: Researchers employ sophisticated models to analyze climate patterns, offering insights into weather extremes and potential future scenarios.
- Sustainable Technologies: The development of renewable energy sources, especially solar and wind, with their efficiency increasing thanks to ongoing research in energy storage materials.
- Policy Formulation: The findings derived from physical science studies inform governmental policies aimed at reducing greenhouse gases. This interaction highlights the necessity for physical scientists to engage with policy advisors to produce actionable frameworks based on their research.
Global Health Crises and Research Directions
The recent global health crises have propelled physical science research into the limelight, emphasizing the necessity for innovative solutions in understanding disease mechanisms and improving public health responses.
- Vaccine Development: The rapid development of vaccines against COVID-19 showcases how physical science, particularly biophysics and material science, plays a role in emergency medicine. Understanding the interaction between proteins and potential vaccines is crucial.
- Data Analysis: Tools developed for data analysis in physical sciences are adapted to track health trends, enabling quicker responses to outbreaks.
- Cross-disciplinary Collaboration: Now more than ever, the blending of physical sciences with epidemiology is vital. Physical scientists find themselves collaborating with biologists, chemists, and health experts, creating forums for dynamic exchange of ideas.
Technological Solutions to Modern Problems
In an era of fast-paced technological advancement, physical science research is at the forefront of addressing pressing modern challenges.
- Smart Materials: Researchers are investigating materials that can self-heal or adapt to environmental conditions, which can lead to sustainable construction practices and a reduction in waste.
- AI Integration: Artificial Intelligence and machine learning algorithms are utilized in data analysis to predict outcomes in various physical sciences fields, from environmental monitoring to medicine, enhancing decision-making processes.
- Global Connectivity: The digital age allows for collaborative projects across borders, breaking down barriers in communication, and enabling global efforts in addressing crises such as climate change and health emergencies.
"The key to resolve these pressing issues lies not only in understanding the science but also in fostering interdisciplinary collaboration across various fields to innovate and implement real-world solutions."
In summary, global issues significantly shape the direction of physical science research, pushing scientists to assume diverse roles that encompass more than just their scientific expertise. They become part of a larger conversation about the future of our planet, demonstrating that science is not an isolated discipline, but inherently intertwined with societal challenges.
Career Paths in Physical Science
The career landscape in physical science research is as diverse as the disciplines themselves. As society faces increasing global challenges, the demand for expertise in physical sciences continues to rise. This section looks at various pathways one may take within this expansive field, shedding light on the implications of each route and what it entails. Understanding these career paths is essential for students, researchers, educators, and professionals seeking to navigate their futures successfully.
Academia and Teaching Opportunities
Pursuing a career in academia is a traditional yet highly valued option for many physical scientists. Here, individuals can become professors, lecturers, or researchers at universities. They not only teach the next generation but also carry out significant research that progresses scientific frontiers. This role is about more than just lecturing; it’s about mentorship, guiding students through challenges, and igniting their passion for learning.
Teaching requires a deep understanding of both the subject matter and the ability to communicate complex ideas in an accessible way. Opportunities in academia often include:
- Conducting Original Research: Engaging in projects that contribute new findings to the field.
- Publishing Scholarly Articles: Sharing research with peers and contributing to academic discourse.
- Attending Conferences: Presenting research and expanding one’s network within the scientific community.
Being in academia can be rewarding, though it often entails a distinct set of pressures. From securing funding for research projects to writing grants, the process can be strenuous and competitive. However, the impact one can make in shaping young minds and contributing to groundbreaking discoveries makes it worthwhile.
Industry and Corporate Roles
Moving from academia to the industry is another viable path for physical science graduates. In industry roles, scientific scholars apply their knowledge directly to product development, quality control, or technical services. Companies ranging from pharmaceuticals to engineering firms are on the lookout for individuals with a strong foundation in scientific principles and practical applications.
In industry, professionals can expect:
- Collaboration with Cross-Functional Teams: Working alongside engineers, marketers, and business professionals to bring scientific innovations to market.
- Real-World Problem Solving: Addressing pressing challenges like product safety, environmental concerns, or optimizing operations.
- Career Mobility: Opportunities for advancement often come with the territory, as companies value employees who can blend scientific expertise with business insight.
The corporate environment may differ significantly from academia in terms of pace, expectations, and profit-driven goals. Those who thrive in dynamic, results-oriented settings may find industry roles fulfilling and lucrative.
Government and Policy Making Positions
Another avenue worth exploring is that of government roles and policy-making. Physical scientists play crucial roles in shaping policies that have far-reaching implications for public health, environmental regulations, and scientific research funding. Government positions can range from research positions in national laboratories to advisory roles in legislative bodies.
Key benefits of pursuing a career in government include:
- Influence on Public Policy: Scientists can advocate for evidence-based policies that promote sustainability, health, and technological advancement.
- Job Stability: Government roles often provide more job security and benefits than private sector positions, which can be a significant draw.
- Interdisciplinary Collaboration: Positions often require working with a variety of stakeholders, including researchers, community groups, and policymakers, to address societal challenges.
However, it's essential to recognize the bureaucratic nature of government positions, which can sometimes impede speedy decision-making.
Overall, each career path in physical science offers distinct advantages and challenges. Engaging in rigorous research, applying knowledge in practical settings, or influencing policy are all avenues that contribute to the rich tapestry of physical science.
Exploring these options can guide individuals to find their niche, allowing them to apply their skills effectively in various contexts of society.
Funding Sources and Research Grants
Securing adequate funding is fundamental for scientific research, especially in the field of physical sciences. Understanding the nuances of funding sources and research grants can make a significant difference in the success rates of projects. Scientific inquiry requires not only time and expertise but also substantial financial resources to facilitate experimentation, data collection, analysis, and dissemination of results. With budgets often stretched thin, exploring various funding avenues is essential for researchers aiming to push the boundaries of knowledge.
Public Funding Opportunities
Public funding represents a major pillar of financial support for physical science research. National institutions such as the National Science Foundation (NSF) and the National Institutes of Health (NIH) allocate funds specifically for scientific inquiries that address essential societal challenges. This type of funding comes with a set of guidelines and approval processes, but it usually offers substantial financial backing.
Consider the following when applying for public grants:
- Eligibility Requirements: Different grants have specific eligibility criteria, which may include the type of institution (e.g., university, non-profit, etc.) and the research focus area.
- Proposal Development: Crafting a compelling proposal is crucial. It should clearly articulate the research goals, potential impact, and the methods implemented.
- Long-term Trends: Awareness of ongoing national priorities can also enhance the chances of securing a grant. Aligning your research with current scientific initiatives may prove beneficial.
Public funding not only comes with monetary support but also lends credibility to the research efforts, helping the scientists earn respect from both peers and the public.
Private Sector Investment
As academic funding becomes more competitive, many researchers look to the private sector as a source of investment. Corporations, particularly those in technology and pharmaceuticals, increasingly aim to fund scientific research that aligns with their business goals. This avenue allows researchers to access essential funds while also fostering partnerships that can lead to innovative projects.
However, collaborating with private investors comes with considerations:
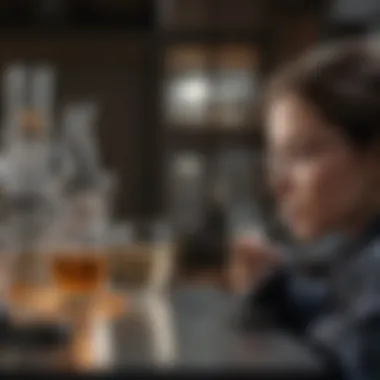
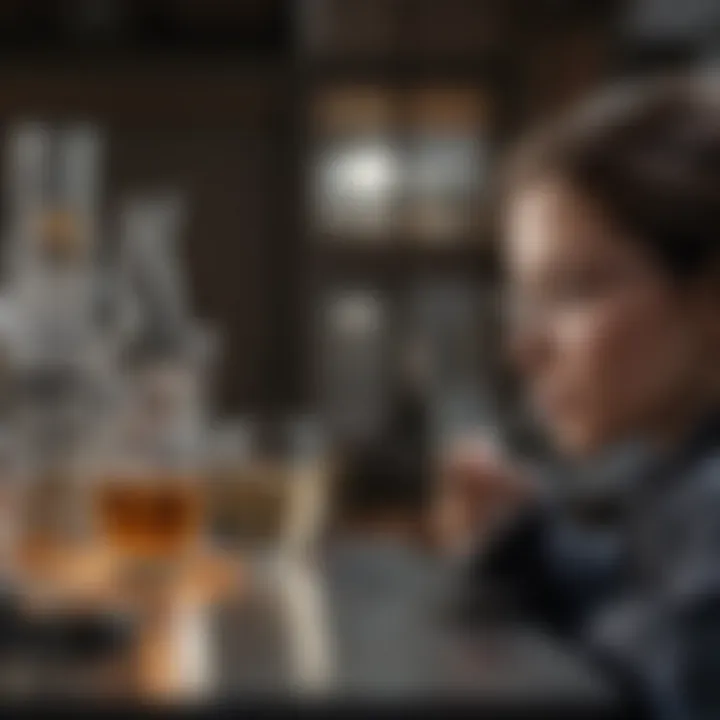
- Research Restrictions: Private companies may impose specific restrictions on the research direction or application of findings, often requiring transparency about how results will be utilized.
- Intellectual Property: Ownership of research discoveries can become a complicated issue. Clear agreements must be in place to determine how intellectual property rights will be shared.
- Reputation Management: Association with a private company can influence public perception of research, so it's imperative that researchers maintain their integrity and prioritize transparency.
In essence, private sector investment can provide vital resources, but navigating the relationship is essential for maintaining research integrity.
International Collaboration and Grants
Exploring funding opportunities beyond national borders can diversify resources for researchers in physical sciences. International collaborations often open doors to grant applications that target global scientific challenges—like climate change or pandemics—that require collective expertise from various countries.
Key aspects of international funding include:
- Diverse Funding Bodies: Organizations such as the European Union's Horizon Europe and global non-profits often provide grants aimed at collaborative research efforts.
- Cross-Country Networking: Establishing connections with international researchers can enhance grant applications, bringing together a blend of skills and perspectives that make a case for funding more compelling.
- Broadened Horizons: Working with international teams fosters exposure to different methodologies and practices, enriching the quality of research outputs.
In a world increasingly interconnected, leveraging international grants not only supports research financially but also encourages the sharing of knowledge and resources across borders.
"The future of physical science research is as much about collaboration and connection as it is about innovation and discovery."
By understanding these funding landscapes—public, private, and international—researchers can navigate the complexities of financial support, enabling them to concentrate on what matters most: advancing the frontiers of physical science.
Case Studies of Noteworthy Research
Case studies serve as a critical bridge between conceptual frameworks and tangible outcomes in physical science research. They provide a detailed glimpse into real-world applications of theories, highlighting the effectiveness, challenges, and innovations that arise in various fields. Analyzing these case studies contributes significantly to understanding key issues and can inspire future research endeavors. Furthermore, they offer evidence-based examples that illustrate complex scientific principles in a relatable manner. The following subsections explore significant case studies that exemplify noteworthy advancements across different sectors in physical sciences.
Innovative Environmental Solutions
The urgent need for sustainable practices has catalyzed research into innovative environmental solutions. One prominent case study involves the development of biochar, a charcoal-like substance produced from organic waste. Researchers began investigating how biochar can enhance soil quality while capturing carbon, effectively addressing two environmental concerns at once: soil depletion and climate change.
The research demonstrated that biochar not only improves soil fertility by retaining nutrients but also assists in sequestering carbon dioxide from the atmosphere. As a result, agricultural practices can evolve to become more sustainable without compromising productivity. This case exemplifies how interdisciplinary approaches—combining biology, chemistry, and environmental science—can lead to impactful solutions.
Breakthroughs in Medical Physics
Medical physics has seen remarkable strides, particularly in the realm of imaging technology. A significant case in point is the development of magnetic resonance imaging (MRI). Early case studies revealed not only how MRI could provide deep insights into human anatomy but also how crucial it is for early diagnosis and treatment monitoring.
For instance, a study evaluated the effectiveness of MRI in detecting brain tumors. The findings showed that MRI could identify tumors much earlier than traditional methods, thus leading to higher survival rates for patients. This advancement underscores the importance of technological evolution and collaboration among physicists and healthcare professionals. Today, MRI remains a cornerstone in diagnostic medicine, illustrating the power of research in shaping healthcare practices.
Advancements in Space Exploration
In the domain of space exploration, the Mars Rover missions constitute a notable case study. The Curiosity rover, which landed on Mars in 2012, has provided invaluable information about the planet's geology and climate. These insights are pivotal in understanding Mars's past and its potential to support life.
The mission highlighted the significance of collaboration in research, as scientists from diverse fields came together to design experiments and analyze data from afar. Curiosity has helped uncover evidence of ancient riverbeds and organic molecules, laying the groundwork for future missions aimed at assessing Mars for possible human habitation.
"These missions not only push the boundaries of technological innovation but also raise fundamental questions about our place in the universe."
By showcasing how scientific inquiry can yield groundbreaking discoveries, these case studies in space exploration exemplify the potential achievements that lie ahead as researchers continue to reach for the stars.
In summary, diving into case studies within physical science research offers essential insights into how theory and practice intertwine. They illuminate pathways for sustainable progress across various sectors, reaffirming the necessity of continued investment and curiosity in advancing physical sciences.
Future Directions in Physical Science Research
As we traverse this intricate landscape of physical science, the importance of understanding its future directions cannot be understated. The realm of scientific inquiry is constantly evolving, shaped by a combination of technological advancements, shifting societal needs, and a relentless quest for knowledge. This section aims to illuminate not just where physical science stands today, but also where it is heading. Addressing potential areas for growth and forthcoming technological predictions will shed light on the evolving dynamics of research in this field.
Potential Areas of Growth
In contemplating future directions in physical science research, several promising areas emerge, each representing unique opportunities for innovation and discovery:
- Biophysics: This interdisciplinary field bridges biology and physics, exploring the physical principles governing biological systems. Progress in this area could revolutionize our understanding of biological processes and lead to novel medical technologies.
- Quantum Physics Applications: Beyond fundamental research, the application of quantum physics in technology is burgeoning. Quantum computing, quantum cryptography, and quantum sensors show great potential, promising computational speeds and security levels unattainable by classical methods.
- Sustainable Materials Science: With environmental concerns on the rise, the development of sustainable materials is crucial. Research focusing on biodegradable plastics, energy-efficient materials, and recycling technologies could significantly impact our ecological footprint.
- Astrobiology: The quest to understand life's existence beyond Earth is gaining traction. As space exploration continues, the integration of physical science with biological and astronomical research is vital to uncover the mysteries of life in the universe.
These areas not only hold immense potential for scientific advancement but also cater to societal needs, making their exploration imperative.
Technological Predictions
Technological foresight plays a pivotal role in shaping the future of physical science research. Below are a few predictions that could define upcoming trends:
- Artificial Intelligence in Research: The integration of AI into research methodologies will likely streamline data analysis, enabling researchers to process vast datasets more efficiently. Machine learning algorithms could assist in predicting experimental outcomes or discovering new materials.
- Advanced Simulation Models: As computational power evolves, so too will the ability to simulate complex physical phenomena. Enhanced simulations might allow scientists to visualize scenarios previously unencountered in experimentation.
- Next-Generation Laboratory Techniques: Automation in laboratories will likely increase efficiency and reproducibility. Innovative tools and robotic systems can perform repeated tasks, leading to increased throughput in experimental research.
"We stand on the threshold of discovery, and our future directions in physical science will illuminate pathways previously shrouded in uncertainty."
- Collaboration Tools: As research becomes increasingly global, the collaboration across boundaries will be supported by improved digital platforms. These tools will facilitate interdisciplinary communication, fostering partnerships that can lead to groundbreaking insights.
By embracing these predictions, researchers can navigate the complex landscape of scientific inquiry, steering their efforts toward impactful discoveries that resonate across society.
In summary, the future of physical science research is not only about the potential for significant breakthroughs but also about addressing pressing global challenges through innovative solutions. Encouraging curiosity and fostering collaborations will be key to unlocking the mysteries that lie ahead.
Culmination
In the dynamic landscape of physical science research, the conclusion serves as a crucial segment that ties together the myriad themes explored throughout the article. It emphasizes not only the significance of the diverse topics discussed but also the interconnectedness of various research fields that contribute to a deeper understanding of the physical world. The reader is invited to reflect on the significance of advancements in materials science, innovations in renewable energy, and the pivotal role quantum mechanics plays in shaping our future.
Summarizing the Importance of Research Themes
The themes addressed in this article reveal the breadth and depth of inquiry within the domain of physical sciences. Each section represents a building block in constructing knowledge, highlighting how even the smallest discoveries can have profound implications. For example, breakthroughs in nanotechnology not only drive forward materials science but also influence the development of new medical technologies and environmental solutions. Moreover, understanding celestial mechanics enhances our grasp of climate patterns and their relation to Earth sciences.
- Integration of Disciplines: The research in physical sciences encourages collaboration across various disciplines, fostering innovation.
- Societal Impact: Themes like climate change and renewable energy showcase the importance of research in addressing global challenges.
- Future Technologies: An emphasis on quantum computing reveals the next frontier in technology, with potential applications in numerous fields.
The themes serve as a reminder of how interconnected research areas can provide multifaceted solutions, drawing from chemistry, physics, and environmental sciences seamlessly.
Encouraging Future Research Initiatives
Looking ahead, there's an undeniable urgency to encourage future research initiatives in physical sciences. With pressing global issues at the forefront, such as climate change, health crises, and resource scarcity, the call for innovative solutions cannot be overstated. It becomes essential to invest in interdisciplinary research efforts that delve into uncharted territories, explore innovative methodologies, and collaborate on a global scale.
"The future of physical science research is not just a matter of inquiry; it's a means of addressing the challenges of today to shape a better tomorrow."
To foster this environment:
- Increase Funding Opportunities: Both public and private sectors should consider expanding their investment in emerging research areas.
- Promote Interdisciplinary Collaboration: Create platforms for scientists from different backgrounds to share insights and data, enhancing the research landscape.
- Develop Accessible Educational Resources: Targeted initiatives could be designed to engage young minds in physical sciences, inspiring the next generation of researchers.
In summary, the physical science research landscape is vibrant and ever-evolving, brimming with potential. By focusing on these key areas and reinforcing the importance of collaboration, we can pave the way for discoveries that not only enrich our understanding but also improve our world.
Citing Important Research Articles
the importance of citing research articles cannot be overstated. Having solid sources backing up your claims enhances not only the clarity of your work, but also its impact amongst peers and the broader community. When highlighting pioneering studies, here are some notable points:
- Attribution: Where credit is due, it's crucial to acknowledge the contributions of past researchers. For instance, a citation of Einstein's pivotal theories in physics can significantly bolster arguments about modern physics.
- Context: Increasing the understanding of a topic often requires a contextual framework. For example, referencing the works of Avogadro or Dalton in discussions on atomic theory sheds light on the historical evolution of that concept.
- Intellectual Property: In many instances, neglecting to cite can lead to accusations of plagiarism. This can tarnish reputations and undermine professional relationships.
Key resources to consider for finding relevant articles include:
- scholarly databases like JSTOR and Google Scholar,
- professional societies such as the American Physical Society, and
- university archives.
Recommended Reading and Resources
Following is a list of recommended resources for those seeking to further delve into the world of physical sciences:
- Textbooks: These often serve as foundational materials. Titles such as "Fundamentals of Physics" by Halliday, Resnick, and Walker provide comprehensive insights.
- Journals: Staying current can be achieved through esteemed journals like "Physical Review Letters" and "Journal of Physical Chemistry" which publish cutting-edge research.
- Web Resources: Websites like Britannica and Wikipedia can offer an initial overview of complex topics.
"The future of physical science lies in collaborative research and a comprehensive understanding of past and present works."