Understanding DNA: Significance and Applications
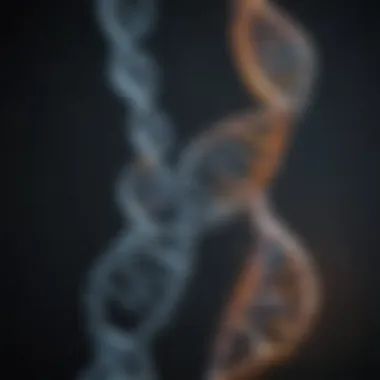
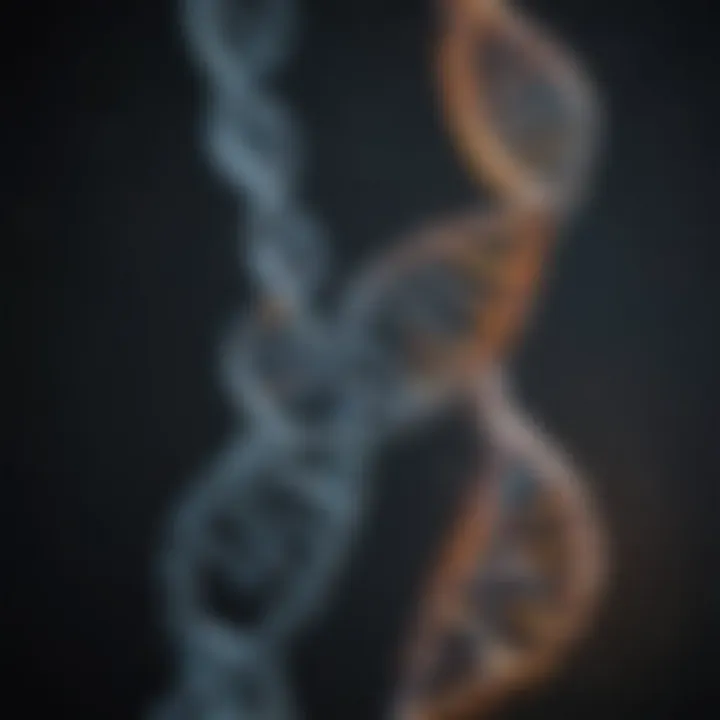
Intro
Understanding DNA is akin to peering into the very essence of life itself. It's the blueprint that governs not only who we are on a genetic level, but also influences our health, behaviors, and ancestry. Although the term DNA might evoke thoughts of intricate lab procedures or cutting-edge genetic technologies, its implications stretch far beyond the confines of science labs. This article seeks to weave together the fundamental aspects of DNA: its structure, its function in organisms, and the nuances of how it shapes our identities and futures.
As we tread along this exploration, we will dissect how DNA plays a pivotal role across various fieldsābe it ancestry tracing, medical diagnostics, or evolutionary biologyāwhile simultaneously confronting the ethical dilemmas that arise from personal DNA analysis.
Key Concepts
Definition of Primary Terms
At its core, DNA, or deoxyribonucleic acid, is a long molecule that contains our unique genetic code. This organic compound is made up of molecules called nucleotides, which are the building blocks of genetic information. Each nucleotide comprises a sugar, a phosphate group, and a nitrogenous base. The arrangement of these basesāadenine, thymine, cytosine, and guanineādictates the instructions that guide every function in a living organism.
Related Concepts and Theories
- Genetics - This is the study of heredity and the variation of inherited characteristics. Understanding genetics is crucial to comprehend how traits are passed from one generation to another through DNA.
- Genomics - A step further, genomics studies the entirety of an organism's genes, thereby broadening our insight into how DNA affects overall biological functions.
- Epigenetics - An exciting field that examines how external factors can influence gene expression without altering the underlying DNA sequence, underscoring the idea that nature and nurture are intricately linked.
"The brilliance of DNA lies not just in the sequence itself, but in the symphony of expression it orchestrates within living beings."
These concepts illuminate various pathways for exploring how DNA impacts not only health but also our behaviors and creativity, creating a tapestry of existence interwoven with genetic threads.
Future Directions
Gaps Identified in Current Research
While much has been uncovered about DNA and its significance, numerous gaps remain. For instance, the role of specific genetic variations in complex diseases is still poorly understood. More research is needed to connect particular DNA alterations with both common and rare health conditions, which could lead to transformative advancements in personalized medicine.
Suggestions for Further Studies
- Ethical Implications of Genetic Data - Investigating how personal genetic information is utilized and protected could reveal much about societal structures and individual rights.
- DNA and Environmental Interactions - There is immense potential in studying how environmental factors influence gene expression over generations, particularly in response to climate change.
- Technological Advancements in Ancestry Research - Further developments in genomic sequencing and analysis tools might offer deeper insights into historical human migrations and lineage tracing.
As we gaze into the crystal ball of genetic research, it is imperative to approach it with both curiosity and caution, balancing scientific exploration with ethical considerations.
Understanding DNA
DNA, or deoxyribonucleic acid, is often referred to as the blueprint of life. Its significance cannot be overstated. In the world of biology and genetics, DNA serves as the foundational molecule carrying the instructions needed for the growth, development, functioning, and reproduction of all known living organisms. Understanding DNA provides insights not just into our biological makeup but also into the broader aspects that influence health, ancestry, and even individuality.
A deep knowledge of DNA permits students, researchers, and healthcare professionals to grasp how genetic information is inherited and expressed. This understanding helps in identifying potential genetic disorders and tailoring medical treatments to individual patient profiles, fostering a more personal approach to healthcare.
Furthermore, the study of DNA opens doors to exploring human evolution, biodiversity, and the complex interactions between genetics and environment. Hence, comprehending DNA enhances our grasp of life itself, revealing connections between different species and the delicate balance of ecosystems.
"DNA is like a book that unfolds the story of life, revealing the chapters of genetics that define who we are."
Definition of DNA
To put it simply, DNA is a long molecule made up of smaller units called nucleotides. Each nucleotide comprises a sugar molecule, a phosphate group, and a nitrogenous base. The sequence of these bases encodes genetic information. Imagine it as a long string of letters, where sequences can create words and sentences, translating into the traits and characteristics that make each organism unique. DNA plays a central role in biological inheritance, where traits are passed down from parents to offspring, encapsulating a legacy that stretches across generations.
Historical Context
The journey of understanding DNA began long before its structure was identified. In 1869, Friedrich Miescher, a Swiss physician, first isolated what he called "nuclein" from the nuclei of white blood cells. However, it wasn't until the early 20th century that scientists started grasping the importance of what they had uncovered. Through a series of experiments and breakthroughs, notably by Alfred Hershey and Martha Chase in the 1950s, it became clear that DNA was the material responsible for heredity.
As research progressed, James Watson and Francis Crick, with the help of Rosalind Franklin's X-ray diffraction images, famously proposed the double helix structure of DNA in 1953. This model elegantly explained how DNA replicates and encodes information. The historical unraveling of DNA has shaped the field of genetics and paved the way for numerous scientific advancements, including genetic engineering and biotechnology.
Understanding DNA is thus not merely an academic pursuit but a journey that continuously reshapes our perceptions of life itself.
Structure of DNA
Understanding the structure of DNA is crucial for grasping its role in genetics and biology. DNA, or deoxyribonucleic acid, carries the genetic blueprint of living organisms. The architecture of this molecule is not just a scientific curiosity; it serves as a foundation for life, affecting everything from heredity to disease susceptibility. By examining how DNA is built, we delve into the pivotal aspects that govern genetic coding, variability, and inheritance.
Nucleotide Composition
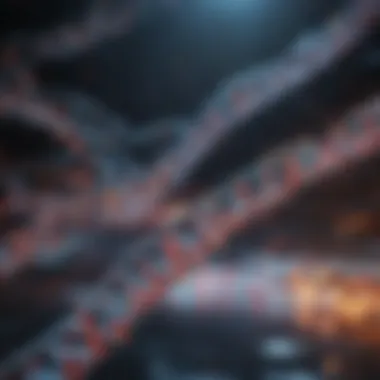
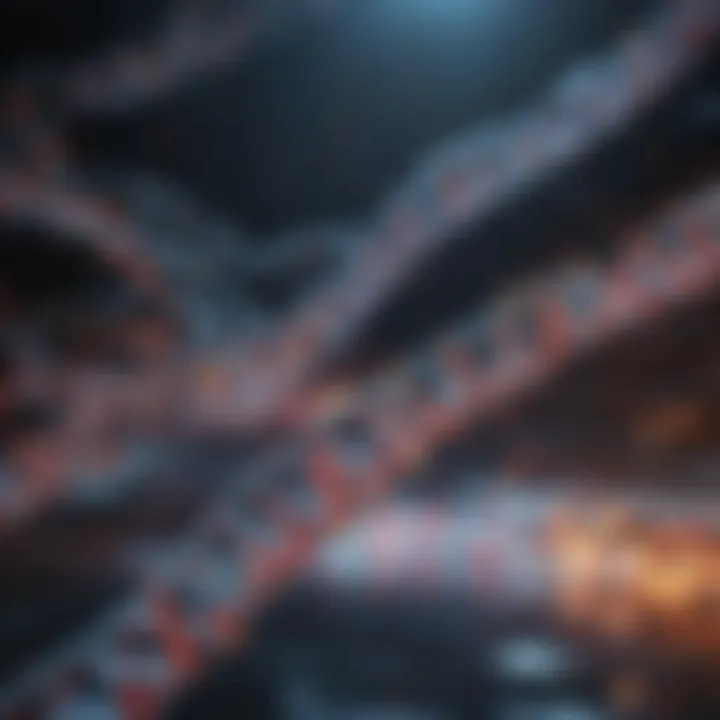
At the heart of DNAās structure lies its basic unit: the nucleotide. Each nucleotide consists of three components: a phosphate group, a deoxyribose sugar, and a nitrogenous base. The nitrogenous bases come in four forms: adenine (A), thymine (T), cytosine (C), and guanine (G). This arrangement of nucleotides forms the genetic alphabet that dictates an organism's traits.
Incredibly, the sequence of these nucleotides, akin to a not-so-simple code, determines everything from eye color to susceptibility to certain diseases. The arrangement and frequency of these bases can also lead to mutations, which in turn can create genetic diversity. This diversity is vital for evolution, enabling species to adapt over generations.
Double Helix Model
The iconic double helix structure, famously elucidated by James Watson and Francis Crick, is perhaps one of the most significant discoveries in biology. Picture a spiral staircase: thatās what a double helix resembles. The structure consists of two strands of nucleotides wrapped around each other, held together by hydrogen bonds between the nitrogenous basesāadenine pairs with thymine, and cytosine pairs with guanine.
This helical shape allows DNA to be packed tightly into the cell nucleus while still being accessible for necessary biological processes like replication and transcription. The stability of this structure is crucial, as it protects the genetic information from damage while enabling the precise copying of DNA during cell division. If it werenāt for this resilience, life as we know it may not even exist.
"The double helix is more than just a structure; it's a storybook of life, telling the tale of how organisms are shaped from generation to generation."
Chromosomal Organization
Going a layer deeper, DNAās organization into chromosomes impacts how genetic information is expressed and inherited. Humans possess 46 chromosomes, arranged in 23 pairs, with one set inherited from each parent. Each chromosome contains a long thread of DNA that is intricately folded and compacted alongside proteins called histones, which help control gene expression and DNA replication.
The way DNA is organized within these chromosomes can influence not just how traits are inherited but also how cells function throughout an organism's life. Various forms of chromatin, which can be tightly or loosely packed, play a direct role in determining which genes are active at any given time. This regulation is not static; it can vary by cell type and environmental factors, showcasing a dynamic interaction between structure and function in genetics.
Through examining the structural composition of DNA, from the individual nucleotides to the overall chromosomal architecture, we lay the groundwork for exploring how genetic information is encoded, expressed, and replicated, ultimately leading to diverse life forms on Earth.
Function of DNA
DNA, or deoxyribonucleic acid, serves as the blueprint for life itself. Its functions are integral to the genetic architecture of all living organisms, affecting everything from development to health. Understanding these functions is essential for appreciating how genetic information is encoded, expressed, and passed on. The intricate processes that govern DNA's roles are pivotal not just for biology but also for medicine, environmental science, and biotechnology.
Genetic Encoding
Genetic encoding refers to the way information is stored within the sequences of nucleotides in DNA. These sequences dictate the synthesis of proteins, which are crucial for the functioning of cells, tissues, and ultimately, entire organisms. Each segment of DNA, called a gene, corresponds to a specific trait or function. The relationship between sequence and function is so precise that even a slight change can lead to significant variations. This encoding is central to genetics, enabling the transmission of traits from parent to offspring. Understanding genetic encoding not only fosters insights into heredity but also opens doors to innovations in gene therapy and personalized medicine.
Protein Synthesis Process
The process of protein synthesis is vital for translating genetic information into functional proteins. This operation is divided into two key steps: transcription and translation.
Transcription
Transcription is the first step in the process of protein synthesis. During this mechanism, the DNA sequence of a gene is copied into messenger RNA (mRNA). This step is a highly regulated process, ensuring that the right genes are activated at the right time. A distinctive aspect of transcription is its reliance on the enzyme RNA polymerase, which binds to the DNA and unwinds the double helix, creating a complementary RNA strand.
The unique feature of transcription lies in its specificity. The cellular machinery precisely identifies which genes need to be expressed, making it a beneficial choice for controlling cellular functions. However, its complexity can also be a double-edged sword; errors in transcription can lead to faulty proteins, contributing to diseases. Despite these challenges, transcription remains one of the most critical processes, as it bridges the gap between the genetic code and protein production.
Translation
Following transcription, translation takes place in the cytoplasm where the mRNA is decoded to synthesize proteins. Ribosomes read the mRNA in sets of three nucleotides, known as codons, each specifying a particular amino acid. The key characteristic of translation is its efficiency and speed, allowing cells to produce proteins quickly as they are needed.
One unique feature of translation is the use of transfer RNA (tRNA), which brings the correct amino acids to the ribosome, matching them with the codons on the mRNA. The advantages of this process lie in its ability to generate proteins rapidly and accurately, crucial for cellular activities and responses. However, translation is not without its pitfalls; misreading the genetic code can lead to the synthesis of nonfunctional or harmful proteins.
Replication Mechanism
The replication mechanism of DNA ensures that genetic information is accurately copied and distributed during cell division. This process is essential for growth, development, and maintenance of all living organisms. DNA replication involves the unwinding of the double helix structure, followed by the synthesis of new complementary strands. Key enzymes, such as DNA polymerase, play a crucial role in this, adding nucleotides one by one to create the new strands.
Ensuring fidelity in this process is critical; any error can have cascading effects, potentially leading to mutations. The accuracy of DNA replication also underpins the stability of genetic information across generations. As research in this area advances, understanding these mechanisms could unlock new treatments for genetic diseases and improve techniques in biotechnology.
DNA in Genetics
Genetics plays a principal role in understanding how traits and characteristics are inherited, and DNA is the cornerstone of this field. It acts not just as a blueprint for living organisms, but also as a narrative of ancestry and a key to comprehending various physiological functionalities. In this context, DNA becomes crucial for several reasons: it helps in predicting certain health risks, provides insights into familial connections, and aids in studying evolutionary patterns. However, the complexity of genetics means there are considerations and implications we must carefully navigate.
Inheritance Patterns
Inheritance patterns refer to the predictable ways traits are passed down from one generation to another. These patterns can be categorized as dominant, recessive, or codominant, and understanding these can demystify why individuals exhibit particular traits or are predisposed to certain conditions. For instance, if both parents carry a recessive gene for a disorder, they have a significant chance of having a child who expresses that disorder.
- Dominant Traits: Simply means that just one copy of the gene is enough for the trait to be expressed. Think of it like a light switch that only needs to be turned on by a single flip.
- Recessive Traits: These require two copies of the gene, much like needing two keys to unlock a door. This is often why certain traits skip generations; they remain hidden when only one parent passes affected genes to their offspring.
- Codominance: A fascinating scenario where both traits are simultaneously expressed. This can be seen in the AB blood type, where both A and B alleles contribute to the final phenotype without one overshadowing the other.
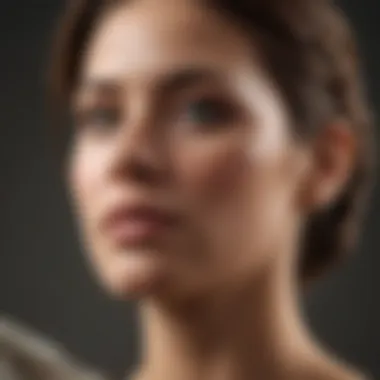
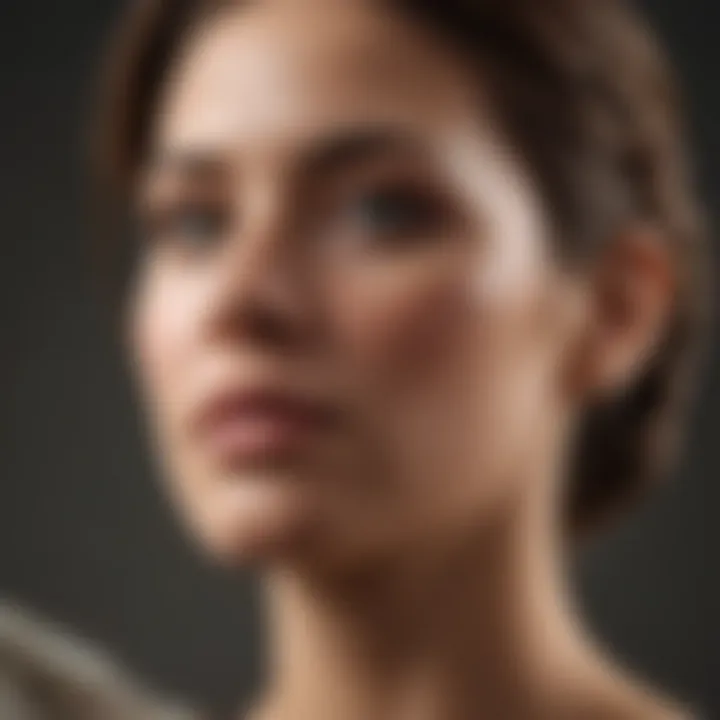
Understanding these patterns guides us to recognize not just individual traits but also the broader implications relating to family dynamics and hereditary health issues.
Mutations and Variability
Mutations are changes that occur in the DNA sequence, and they can happen for a multitude of reasons including environmental factors, errors during DNA replication, or even simply due to chance. While often regarded with a negative connotation, mutations are an essential part of genetic diversity.
- Beneficial Mutations: From an evolutionary perspective, some mutations can provide an advantage. They contribute to adaptability, like bacteria developing resistance to antibiotics. This resilience permits survival in changing environments.
- Neutral Mutations: These occur without an immediate effect on the organism's fitness. They might not alter anything at all or can provide variations with no significant advantage or disadvantage. Such mutations, over time, can accumulate and shift genetic diversity.
- Harmful Mutations: Unfortunately, some mutations lead to disorders or dysfunctional proteins, which could severely affect an individual's health. For example, sickle cell disease arises from a single point mutation but provides a malaria resistance advantage in some populations.
The balance of beneficial and harmful mutations is the driving force behind evolution, highlighting the dynamic nature of DNA.
Genetic Disorders
Genetic disorders offer a deep dive into how DNA translates into real-world health scenarios. They arise from abnormalities in one or more genes and can stem from a wide range of mutations. Knowing about these conditions is essential for diagnosis and treatment, shedding light on implications for individuals and their families.
Common types of genetic disorders include:
- Single-gene Disorders: Conditions like cystic fibrosis or Huntington's disease, caused by changes to a single gene, can provide predictable inheritance patterns.
- Chromosomal Disorders: These arise when there is an abnormal number or structure of chromosomes. Down syndrome, caused by the presence of an extra chromosome, is a primary example that illustrates how genetic makeup can manifest in observable traits.
- Multifactorial Disorders: These involve a combination of genetic, environmental, and lifestyle factors. Conditions like heart disease or diabetes don't have a single genetic link, making them complex to analyze but vital in understanding overall health risks.
Understanding these disorders influences public health approaches, genetic counseling, and research aimed at treatment and prevention strategies. The implications reach far beyond individual cases, affecting family planning, societal health resources, and even insurance policies.
Applications of DNA Knowledge
Understanding the applications of DNA knowledge is crucial as it impacts various sectors including genetics, medicine, and law enforcement. In todayās rapidly advancing world, harnessing DNA information is not just a scientific endeavor but increasingly part of our daily lives. By exploring the practical usages of DNA, we can appreciate both the potential benefits and challenges that arise. This perspective is pivotal, especially when considering personal identity, legal matters, and medical advancements.
Ancestry and Genetic Heritage
One of the most fascinating applications of DNA is its ability to trace ancestry and uncover genetic heritage. By analyzing specific markers within an individual's DNA, companies like AncestryDNA and 23andMe provide insights into familial lineages and ethnic backgrounds. These genetic insights can sometimes illuminate stories of migration and historical origins that families might not have been aware of.
When you send in a sample, often saliva, laboratories extract your DNA and compare it with extensive reference databases. The results can show which regions of the world your ancestors might have hailed from. This journey into your past can be quite revelatory; people often learn they are part of a kinship that spans continents. Furthermore, such findings can foster stronger family bonds as relatives connect through shared genes they didn't know they had.
However, while tracing back roots can be fulfilling, it's essential to tread carefully. Thereās a potential for unexpected surprises. For instance, discovering a different parentage than assumed could shake the very foundation of oneās family narrative.
Medical Diagnostics
The field of medical diagnostics has seen remarkable changes thanks to DNA research. Understanding the genetic basis of various diseases is paving the way for tailored treatment plans that are more effective and efficient. For example, the identification of mutations in genes like BRCA1 and BRCA2 can significantly influence cancer risk assessment. Medical professionals can now provide preemptive measures and personalized screening options based on an individualās genetic profile.
Moreover, developments in genomic medicine enable the examination of patient genomes to find predispositions to various conditions. This attention to a personās unique genetic makeup can inform not only treatment but also lifestyle changes before illnesses manifest.
āThe recognition of genetic variants offers a beacon of hope for diagnosing and managing complex diseases.ā
However, this raises ethical concerns about genetic privacy and discrimination. Knowing one's risks could lead to heightened anxiety or even discrimination in insurance and employment. Such realities make it imperative for society to consider regulations that protect individuals.
Forensic Science
The role of DNA in forensic science cannot be overstated. DNA profiling has revolutionized how law enforcement tackles crime, leading to more accurate identifications of individuals involved in criminal activities. Collecting DNA samples from crime scenes, such as hair, blood, or skin cells, allows forensic experts to compare them against suspect databases for matching.
This method has not only solved countless cases but has also exonerated individuals wrongfully convicted. Famous cases, like the exoneration of individuals through DNA evidence, reflect the potential for a more just legal system enabled by scientific advancements.
However, the use of DNA in forensics isnāt without controversy. Misinterpretations or errors in the lab can lead to serious misjudgments. Moreover, ethical dilemmas arise when considering how much personal DNA data should be stored and who gets access to it. Balancing justice and individual rights will be a continuing challenge.
Ethical Considerations in DNA Research
As we delve deeper into the wonders of DNA, we cannot overlook the accompanying ethical considerations that emerge in the realm of genetic research. The impact of these considerations stretches far beyond laboratories and research papers; they resonate throughout society, influencing policies, personal choices, and our very understanding of identity.
Privacy Concerns
One of the most pressing ethical considerations revolves around privacy. When individuals participate in DNA testing or research, they often provide access to their genetic information, raising questions about how this sensitive data is stored and who gets to see it. The fear looms that this information could fall into the wrong hands, leading to potential misuse. In recent years, weāve witnessed a growing concern about data breaches in various industries. Imagine a world where your genetic predispositions, such as susceptibility to diseases or inherited traits, are accessible to employers or insurance companies. This really puts a spotlight on the need for robust regulations that protect individuals' genetic data.
"The right to genetic privacy is as important as the right to physical privacy. Our genetic code is the essence of who we are, and it deserves protection."

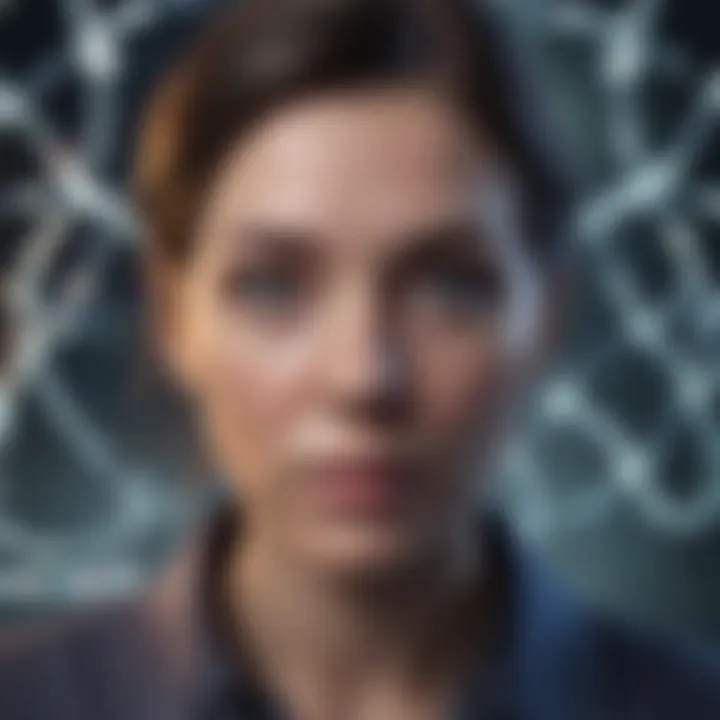
Genetic Discrimination
Genetic discrimination refers to the unequal treatment of individuals based on their genetic information. This issue raises a crucial ethical dilemma: Should individuals be judged or treated differently based solely on their genetic predispositions? The reality is that genetic tests can reveal a person's potential for various health conditions, which might lead employers or insurers to make biased decisions. For instance, if an insurance provider discovers a person's likelihood of developing a chronic illness, they might charge higher premiums or deny coverage altogether. Such practices could perpetuate inequalities and foster environments of fear and mistrust. Regulatory frameworks need to evolve to combat this issue, ensuring that genetic information does not become a tool for discrimination.
Ownership of Genetic Information
Who truly owns oneās genetic information? This is a question that has sparked debates among ethicists, scientists, and the general public alike. When individuals submit samples for testing or research, do they relinquish all rights to their genetic data? Often, research institutions and biotech companies might claim ownership over the data derived from these samples. This raises the question of whether individuals should have say over how their genetic information is used, especially in research that could lead to commercial profit. People have a right to know how their data is utilized, and they should have control over whether to share their information for research or commercial use. This aspect of ethical consideration not only impacts individuals but also the trajectory of medical research as a whole, guiding how trust is built in the scientific community.
In summary, ethical considerations in DNA research are multifaceted and critical. As we progress in understanding and utilizing DNA's power, addressing these ethical challenges is essential for fostering a responsible and equitable approach to genetic research and its applications. The relationship between individuals and their genetic data requires careful navigation, blending science with ethical integrity.
Current Trends in DNA Research
In the rapidly evolving field of genetics, understanding the current trends in DNA research is crucial for grasping the potential directions in which this science may head. As researchers uncover more about the functional dynamics of DNA, several trends stand out that promise to reshape both medical practices and our conceptions of genetics.
CRISPR Technology
One of the most revolutionary developments in modern genetics is the advent of CRISPR technology. Standing for Clustered Regularly Interspaced Short Palindromic Repeats, this system allows for precise edits to DNA sequences. Through a mechanism that mimics the way bacteria fend off viruses, CRISPR enables scientists to cut and modify genetic material at specific locations.
The importance of CRISPR can hardly be overstated. It opens up pathways for treating genetic disorders that were once thought incurable. Conditions like sickle cell anemia or certain types of genetic blindness are being targeted using this groundbreaking technology. Moreover, the ease and cost-effective nature of CRISPR compared to earlier methods make it accessible to a wider range of research initiatives and applications. However, this power does come with a slew of ethical questions, particularly regarding its use in germline editing.
Genomic Medicine
Alongside CRISPR, genomic medicine is emerging as a vital area of exploration. This branch aims to harness the data derived from a personās complete set of DNA to personalize medical treatments. In practice, this means that healthcare can evolve from a "one-size-fits-all" approach to a tailored methodology, with treatments informed by individual genomic profiles.
The benefits of genomic medicine are significant:
- Prevention: Identifying genetic predispositions enables targeted prevention strategies.
- Tailored treatments: Medications can be adjusted based on genetic makeup, improving efficacy and reducing side effects.
- Early diagnosis: Genomic tests can lead to the identification of diseases before symptoms manifest, allowing for earlier intervention.
Nevertheless, this trend also brings challenges, particularly concerning privacy and data security. Keeping genomic data safe, while also empowering patients with their information, remains a significant hurdle for researchers and healthcare providers alike.
Synthetic Biology
The realm of synthetic biology represents another exciting frontier in DNA research. This field combines biology and engineering to design and construct new biological parts, devices, and systems. Synthetic organisms can be programmed to perform useful tasks, like producing biofuels or medicines, in remarkably effective ways.
The implications of synthetic biology are vast:
- Biomanufacturing: This approach allows for the creation of sustainable, bio-based materials that reduce dependence on fossil fuels.
- Environmental applications: Genetically modified organisms can be developed to clean up pollution or monitor environmental changes.
- Agricultural innovation: With the aid of synthetic biology, crops can be engineered for better yield and resilience against pests and diseases.
As the line between natural and engineered life blurs, it raises important philosophical and ethical debates about our role in manipulating life systems.
"The future of DNA research lies not just in understanding the code of life but also in rewriting it to meet the challenges we face today and in the future."
In summary, the trends of CRISPR technology, genomic medicine, and synthetic biology mark a new era in DNA research. Each avenue presents significant advantages and challenges, weaving a complex tapestry of opportunities in health care, environmental sustainability, and beyond. Navigating these waters with awareness and responsibility will be integral to leveraging their full potential.
The Future of DNA Studies
As we look ahead, the landscape of DNA research seems to be brimming with possibilities. The understanding of DNAās role extends to various fields, from healthcare to social structures. With rapid advancements in technology and methodologies, the importance of these studies is unmistakable. The following sections delve into how these developments may shape the future, focusing on health care impacts, social implications, and the challenges that lay on the horizon.
Impact on Health Care
The implications of DNA studies in health care canāt be overstated. The potential for personalized medicine is on the rise, revolutionizing how diseases are treated and prevented. Tailoring treatments based on individual genetic profiles means that therapies could be more effective and carry fewer side effects.
- Genomic Testing: By analyzing an individualās DNA, healthcare providers can identify predispositions to certain diseases, allowing for proactive measures. This brings about a paradigm shift; prevention is better than cure, as they say.
- Targeted Therapies: The days of a one-size-fits-all approach to medication may soon be behind us. Targeted therapies are already being designed to attack specific genetic mutations, offering hope for patients with conditions like cancer.
- Gene Therapy: While still in its infancy, the techniques for gene editing, particularly CRISPR, lead the charge in addressing genetic disorders. This could mean not just treating symptoms but potentially eradicating conditions altogether.
"The future of medicine is in our genetic makeup, offering customized treatments that resonate with each individualās unique blueprint."
Social Implications
The rise of DNA technology also presents various social implications worth noting. Understanding our DNA can impact societal constructs, from identity to privacy concerns. Here are some key points:
- Identity and Belonging: Knowledge of ancestry and genetic background helps individuals relate to cultural heritage. It raises questions about identity in multicultural societiesāhow do we define ourselves when genetic information provides a broader picture?
- Access and Inequality: As these technologies become available, thereās a risk of access becoming a privilege of the few. Socioeconomic factors could create a gapāthose with resources may benefit more from advancements in genetic understanding.
- Informed Citizenship: With individuals becoming more aware of their genetic potential, they may engage more critically with topics such as public health initiatives, leading to more informed citizenship and societal participation.
Challenges Ahead
Despite the optimistic outlook, navigating the future of DNA studies poses its own set of challenges. Addressing potential hurdles is essential to maximize benefits while minimizing risks. Consider:
- Ethical Considerations: The ability to edit genes brings ethical dilemmas. How far should we go in altering genetic traits? The boundaries between therapy and enhancement remain blurred.
- Privacy Issues: With genomic data being sensitive, privacy concerns are paramount. The handling and storage of this information raise questionsāwho owns genetic data, and how can we protect it?
- Regulatory Standards: As studies ramp up, clear guidelines on the use of genetic data and technologies are crucial. Crafting policies that protect individuals while fostering innovation is no small feat.